…they aren’t where you would think. ASF fellow Dr. Donna Werling from UCSF explains.
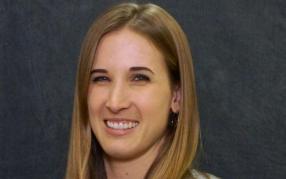
Dr. Donna Werling studies sex differences in autism at UCSF
Males are approximately 4 times more likely to be diagnosed with autism spectrum disorder (ASD) than girls and women1,2, and for the most part, scientists don’t know why. To figure out how sex differences in human biology might contribute to ASD risk, we compared patterns of gene expression from males and females in the developing and adult human brain. We found that genes that show higher expression levels in males relative to females include many of the same genes that are expressed at higher levels in the brains of individuals with autism relative to controls3. What these male-elevated and ASD-elevated genes do in the brain may be a clue to the sex difference in ASD prevalence.
A closer look at these genes showed that they are known to be involved in the functions of microglia and astrocytes. Microglia are a type of cell involved in the immune system of the brain, and astrocytes are different type of cell that provides support to neurons that communicate across regions of the brain. Our findings suggest that these non-neuronal cell types may play a role in the biology behind the male-biased risk for ASD and may provide insight on why more males are diagnosed compared to females.
How did we do this? It involved lots of time and access to valuable resources.
In order to understand the role of different cell types in the brain, we needed to study actual brain tissue from people. In order to do this, we turned to a project called BrainSpan4, a publicly available data set generated from post-mortem human samples from the brains of males and females. Brain tissue is an incredibly rare resource and this dataset is invaluable in our research. Using these data from BrainSpan, we generated a list of genes that have different expression levels in males and females in the adult cerebral cortex and we used this list to look at two questions relating to autism:
Our first question was “Do genes that are directly associated with autism risk differ between males and females?” For example, the genes CHD8 and TSC1 which are both known to contribute to the cause of ASD5,6. If these genes show up differently in males and females, then mutations in these genes would affect male and female brains differently, which might lead to the sex differences we see in ASD diagnoses. In fact, we found this was not the case: genes directly associated with autism risk do not show up differently in males and females.
Our second question was “Do genes that are indirectly associated with autism differ between the sexes?” For our study, we considered genes that show changes in expression level in the ASD brain to be indirectly associated with autism7,8. This includes genes that show low expression in the ASD brain; these genes may be affected by directly associated ASD risk genes from our first question. This also includes genes that show high expression in the ASD brain; these genes are related to the brain’s immune system. If these indirectly associated genes with altered expression in the ASD brain are expressed differently in males and females, this would suggest that autism and sex-differential biology affect some of the same molecular or cellular pathways in the brain. These shared pathways may modulate the impact of risk factors such that males are more strongly affected. In contrast to our first question, we found that several of the indirectly ASD associated gene sets did overlap significantly with sex-differentially expressed genes. Specifically, gene sets with coordinated, elevated expression in the ASD brain overlap with genes with higher expression in males, and these overlapping genes are associated with microglia and astrocytes.
These results suggest that, instead of directly affecting ASD risk genes, sex-differential biology interacts with ASD risk biology downstream from these ASD risk genes, and that this interaction may involve the functions of microglia and/or astrocytes.
We repeated our experiment and replicated our results in two additional data sets, including one data set from prenatal brain samples. This is especially important because midfetal development is a key time for the expression, and presumably function, of directly associated ASD risk genes10,11. Therefore, the patterns that we see demonstrate that not only do microglial and astrocyte genes differ by sex in the adult brain, but they also differ in the same region and time window of development when ASD risk genes are robustly expressed..
That microglia and astrocytes may be involved in sex differences in ASD is intriguing, since these types of cells are becoming more and more recognized for their roles in neural circuitry development by supporting the formation, maintenance, and/or pruning of synapses12,13. Therefore, sex differences in the functions of these cell types could lead to differences in neuroanatomy and function between males and females. Supporting this idea, studies in rodent models show that the numbers of microglia that take up residence in the brain during early development differ between males and females14, and also that astrocytes in the hypothalamus express estrogen receptors and change morphology in response to estradiol exposure15. However, it is not currently known whether these phenomena also occur in humans.
Since microglial and astrocyte genes and cells function downstream from currently known ASD risk genes, treatments that mimic these specific processes could potentially help large numbers of patients with diverse genetic backgrounds. However, several key questions remain. First, our results must be replicated in larger data sets, preferably including comparable numbers of typically developing and autistic males and females. This will be necessary to corroborate the patterns we see and to more directly investigate the interacting effects of sex and ASD on gene expression. The only way we can do this is with more brain tissue from people with autism, which is why the Autism BrainNet is so important for the community. Further research will also be required to determine why microglial and astrocyte genes differ in males and females, and to clarify exactly how sexually dimorphic microglia and/or astrocytes function in the brain to influence neurodevelopment and ASD risk.
Eventually, a major goal will be to translate these findings into tangible benefits for individuals with ASD and their families, perhaps by developing therapeutics that target functional pathways at the intersection between sexually dimorphic and autistic biology. By implicating ASD-upregulated microglial and astrocyte genes at this intersection, our study has uncovered an intriguing new avenue to pursue in our endeavor to fully understand ASD and the striking sex difference in its prevalence.
We gratefully acknowledge the data resources of the BrainSpan consortium, as well as the generous donations from individuals and families that make such invaluable data sets possible. I also acknowledge my co-authors on this study, Dr. Daniel Geschwind and Dr. Neelroop Parikshak, our funding from the US National Institute of Mental Health (5R37MH060233 and 5R01MH094714 to DHG; Autism Center for Excellence network grant 9R01MH100027; F30MH099886 and T32MH073526 to NNP; F31MH093086 to DMW), and Dr. Stephan Sanders and Dr. Alycia Halladay for their input on this post. Finally, I would like to thank the Autism Science Foundation for the opportunity to share this work with the community.
References
- Wingate, M. et al. Prevalence of autism spectrum disorder among children aged 8 years – Autism and Developmental Disabilities Monitoring Network, 11 sites, United States, 2010. Morbidity and Mortality Weekly Report Surveillance Summaries 63, (2014).
- Fombonne, E. Epidemiology of pervasive developmental disorders. Pediatr. Res. 65, 591–598 (2009).
- Werling, D. M., Parikshak, N. N. & Geschwind, D. H. Gene expression in human brain implicates sexually dimorphic pathways in autism spectrum disorders. Nat. Commun. 7, 10717 (2016).
- BrainSpan: Atlas of the Developing Human Brain. (2013). at <" rel="nofollow">http://www.brainspan.org>
- Basu, S. N., Kollu, R. & Banerjee-Basu, S. AutDB: a gene reference resource for autism research. Nucleic Acids Res. 37, D832–6 (2009).
- Iossifov, I. et al. The contribution of de novo coding mutations to autism spectrum disorder. Nature 515, 216–221 (2014).
- Voineagu, I. et al. Transcriptomic analysis of autistic brain reveals convergent molecular pathology. Nature 474, 380–384 (2011).
- Gupta, S. et al. Transcriptome analysis reveals dysregulation of innate immune response genes and neuronal activity-dependent genes in autism. Nat. Commun. 5, 5748 (2014).
- Darnell, J. C. et al. FMRP stalls ribosomal translocation on mRNAs linked to synaptic function and autism. Cell 146, 247–261 (2011).
- Willsey, A. J. et al. Coexpression networks implicate human midfetal deep cortical projection neurons in the pathogenesis of autism. Cell 155, 997–1007 (2013).
- Parikshak, N. N. et al. Integrative functional genomic analyses implicate specific molecular pathways and circuits in autism. Cell 155, 1008–1021 (2013).
- Stevens, B. Neuron-astrocyte signaling in the development and plasticity of neural circuits. Neurosignals 16, 278–288 (2008).
- Schafer, D. P. et al. Microglia sculpt postnatal neural circuits in an activity and complement-dependent manner. Neuron 74, 691–705 (2012).
- Schwarz, J. M., Sholar, P. W. & Bilbo, S. D. Sex differences in microglial colonization of the developing rat brain. J Neurochem 120, 948–963 (2012).
- McCarthy, M. M., Todd, B. J. & Amateau, S. K. Estradiol modulation of astrocytes and the establishment of sex differences in the brain. Ann. N. Y. Acad. Sci. 1007, 283–297 (2003).