- You can use TENs machines to prevent muscle loss whilst injured
- Nutrition is important – Under-eating will lead to more muscle loss
- Clenbuteral is an illegal performance enhancing drug that prevents muscle loss
- Alpha Lipoic Acid supplementation may help prevent muscle loss and help to alleviate some of the effects of the loss of insulin sensitivity
- No significant de-training effects occur if you can get back to training within 2 weeks
For information purposes only. Exercise at your own risk
Professional sport in the twenty first century has evolved into a competitive business. Immense financial investments into sports clubs, sports governing bodies and development centres, has seen athletes converted into commodities that are expected to deliver success. As sports clubs float on the stock market, athletes follow rigorous training regimes that push them to their physical and mental limit. MMA shares the pressures and expectations of any other top-level sport. As it verges on the brink of mainstream acceptance, it survives primarily as a form of entertainment, rather than participatory sport.
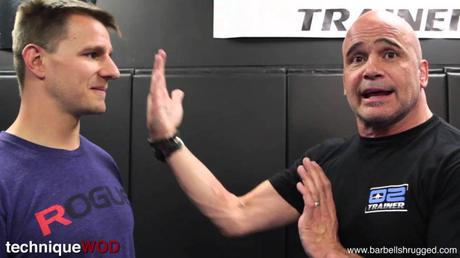
Pay per view and DVD sales underpin its survival, and as a result the combatants are expected to display feats of awesome athletic ability and technique, each time they enter the cage. Fuelled by massive economic investment, MMA training has developed into a scientific discipline that addresses and attends to every aspect of physical and mental fitness.
However, as boundaries in performance are continually broken and re-established, athletes increase their risk of ‘burnout’ or physical injury. Although the recruitment of sports psychologists and physiotherapists may reduce the likelihood of such an event, it is doubtful that a competitor will end his/her career without having to endure a serious injury or slump in performance at some point. Successful fighters find a balance between hard training and rest.
Rest is required so that adaptation to training may occur. Progression and consistency is key in any training schedule and injuries can set back athletes by months, even years. It is therefore crucial that athletes limit their risk of injury by avoiding over-training, and by acquiring knowledge concerning how best to limit the ‘detraining’ effect, so that an injury or rest period is managed correctly. The following report will examine the consequences of rest and injury upon different aspects of athletic performance, and will also discuss the best ways of inhibiting the detraining effect. A number of reports and research documents shall be examined and critiqued, so that by the end of the report the question ‘detraining: threat or therapy’ will be successfully discussed and answered.
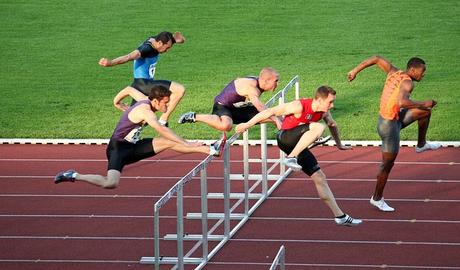
Injury & Deconditioning
According to Quinn (2004), one of the five principles of conditioning is the ‘Principle of Use/Disuse’. Simplified, this would imply that your muscles hypertrophy with use and atrophy with disuse. However, this ‘rule’ should not be taken completely literally, as it is important to find a balance between training and rest. There must be periods of low intensity between periods of high intensity to allow for recovery and training adaptation to occur.
Deconditioning will occur with long periods of rest. How quickly an individual loses fitness depends on how fit he/she is, how long you he/she has been training and how long he/she has stopped training (http://sportsmedicine.about.com/cs/exercisephysiology/a/aa073003a.htm; Willmore & Costill, 1999; Davis, Bull, Roscoe & Roscoe, 1999).
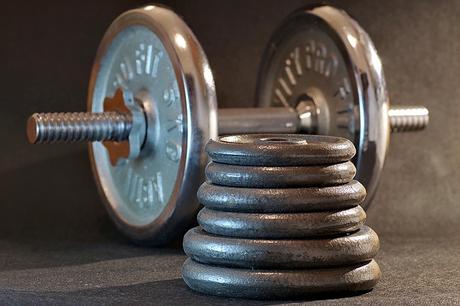
Thanks to recent research, deconditioning theories have become more clear and specific. Mujika et al (2000) studied well-conditioned athletes who had trained for year and then stopped exercise all together. After a three month rest from cycling training, researchers found that the athletes lost 57% of their aerobic conditioning.
However, Rietjens et al (2001) investigated the effects of reduced training on physical condition and performance in well-trained cyclists and whether an intermittent exercise programme would maintain physiological training adaptations.
Neither group showed changes in maximal workload for the Continuous Training and Intermittent Training group respectively, and it was concluded that well trained cyclists who reduce training intensity and volume for 21 days can maintain physiological adaptations, as measured during submaximal and maximal exercise. In addition the study seem to suggest that an intermittent training regimen has no advantage over a continuous training regimen during a detraining period.
It would appear that a certain level of exercise is required to maintain sport specific fitness.
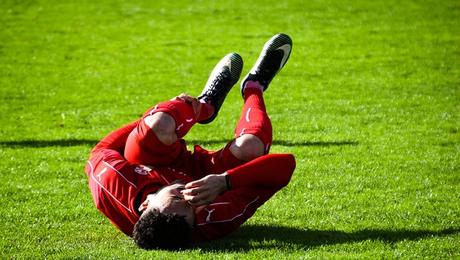
However, the intensity and duration of this ‘level of activity’ remains controversial and is specific to each sport. The level of activity necessary will depend upon an athlete’s baseline fitness level and sport (Winters & Snow, 2000). However, it would appear that if an athlete can maintain some exercise on a weekly basis, he/she could retain a greater percentage of his/her baseline fitness levels. Although it is not always possible, athletes should attempt to adapt their training in accordance to an injury.
Cross training through an injury is always an option, for example ‘aqua-jogging’ is an effective activity that enables runners to maintain fitness levels whilst recovering from repetitive strain injuries to the knees, shins and/or back (http://sportsmedicine.about.com/cs/exercisephysiology/a/aa073003a.htm). Repetitive strain injuries are increasingly common among top athletes (Smith, 2003). Many top athletes train 3-5 hours per day in an attempt to improve their level of conditioning and skill.
Physical activity is a way of life for these people, and can dominate their thoughts and actions for a number of years. When an individual stops participating in high levels of physical activity, the physiological changes that occur are usual referred to as ‘detraining’. This often occurs when an athlete becomes injured. Swain et al (1994) and Cooper (1982) suggest that a few days of rest or inactivity may enhance performance and physiological adaptations to training. However there is a critical, individual specific, period of rest, where performance capability begins to decrease. It would also appear that different aspects of fitness deteriorate at different rates, and all levels of detraining depend of training history. For example, within a few days, a person’s insulin sensitivity is decreased, whereas muscular strength can take weeks to deteriorate (Taafe & Marcus, 1997).
Skeletal Muscle Size, Strength & Power
Sports such as powerlifting and rugby predominantly involve short, explosive movements. However most sports, such as wrestling require high levels of endurance and several other specific types of fitness and skill. Strength training may therefore, not always remain as a top priority, and may have to be maintained whilst other aspects of performance are targeted for improvement. Haggmark et al (1986) suggest that continuing to train once every 10 to 14 days, athletes can maintain strength and power for up to 4 months.
This may be important for the injured athlete, who cannot train at maximal intensity. The real losses in muscle mass and strength occur, not when training per se is stopped, but when a joint is completely immobilized. Daily activities are enough to reduce any sudden loss in performance capacity, and inhibit the drastic losses that occur through complete immobilisation.
Skeletal muscle undergoes a substantial decrease in size once a certain limb or area of the body becomes inactive. This is known as atrophy, and is accompanied by a considerable loss in power and strength. Total inactivity results in rapid losses, whilst prolonged periods of reduced activity can result in more gradual losses that become quite significant.
Taaffe and Marcus (1997) investigated the effects of cessation (8 weeks) and subsequent resumption (12 weeks) of training on muscle strength in elderly men, after completing 24 weeks of resistance training. The results indicate that elderly men lose some muscle strength following short-term detraining, but that only a brief period of retraining suffices to regain maximal strength. Reduction of fiber cross-sectional area with detraining, suggests that much of the retention in strength with detraining and reacquisition of lost strength with retraining reflects neural adaptation.
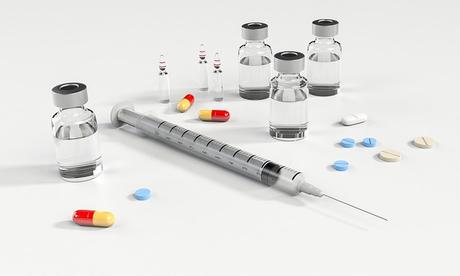
The strength that was maintained could be accounted for by neuro-muscular adaptation that occurs in the first weeks of adoption of a weight training programme; this relates to the acquisition of technical correctness and the ability to recruit the maximal number of muscle fibres with correct timing. One of the very first studies to investigate detraining (McMorris et al, 1954) showed that 45% of strength gains from a 12 weeks resistance training programme, were maintained following a 1 year absence from training. It has been suggested that this may also reflect retention of neuromuscular functioning (Housh et al, 1995). A study conducted by Costill et al (1998) upon university swimmers showed that a 4 week rest from activity did not affect shoulder or arm strength, however, swimming power was reduced by approximately 10% whether the swimmers underwent complete rest or trained at a reduced frequency of one session per week.
This suggests that functional strength and/or power may be reduced at an accelerated rate compared with strength per se. This study points to a possible area of inaccuracy in other studies – measurement techniques. The strength was measured ‘on land’ with a biokinetic swim bench, whilst power was measured in the water, using tethered swimming that allows the swimmers to use natural actions. It would appear therefore that the less specific land measurements may not reflect any effect upon performance.
When muscles are not used in the specific way that accurately replicates performance, neurological control appears to be reduced (GSSI, 2001 Conference) and normal fiber recruitment is hindered. Rest is an essential component of strength training which allows for muscular adaptation to a training load. Strength can be maintained a lot easier than it is acquired (Haggmark et al, 1986). Coaches and trainers should take this into account when designing periodised macro cycles which are tailored to the specific needs of a sport. Fitness tests such as the standing jump test and varies one repetition max tests should be used at intervals during the season in order to monitor fitness levels to ensure that each aspect of conditioning is either being maintained or improved.
Muscular Endurance
Muscular endurance decreases after just 2 weeks of inactivity (Winters & Snow, 2000). Skeletal muscle is characterized by its ability to dynamically adapt to variable levels of functional demands. During periods of insufficient training stimulus, muscular endurance decreases rapidly. Initial deterioration of endurance may be due to a decreased capillary density, which could take place within 2–3 wk of inactivity. Arterial-venous oxygen difference declines if training stoppage continues beyond 3–8 wk. Rapid and progressive reductions in oxidative enzyme activities bring about a reduced mitochondrial ATP production (Winters & Snow, 2000). The above changes are related to the reduction in VO2max observed during long-term training cessation.
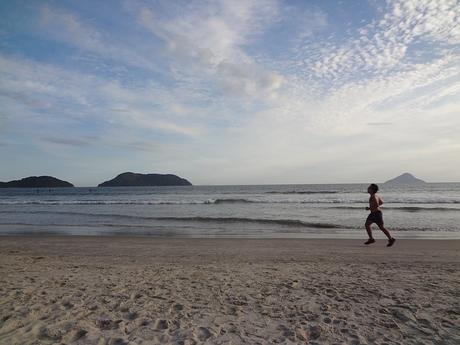
These muscular characteristics remain above sedentary values in the detrained athlete but usually return to baseline values in recently trained individuals. Glycolytic enzyme activities show non-systematic changes during periods of training cessation. Fibre distribution remains unchanged during the initial weeks of inactivity, but oxidative fibres may decrease in endurance athletes and increase in strength-trained athletes within 8 wk of training stoppage. At this time, not enough evidence is available to determine, whether this performance decrement results from changes in the muscle or from changes in cardiovascular capacity (Coyle, 1984). Studies have shown that after a week of cast immobilisation, the activities of oxidative enzymes such as succinate dehydrogenase and cytochrome oxidase decrease by 40 to 60%. In contrast, when athletes stop training, the activities of the muscles’ glycolytic enzymes, such as phosphofructokinase, change little, if at all, for at least 4 weeks.
Coyle et al (1984) observed no change in glycolytic enzyme activity with up to 84 days of inactivity, but an average fall of over 50% in the activity of oxidative enzymes. Despite the apparent resilience of glycolytic enzymes to detrimental effects of detraining, muscle glycogen content decreases. This may be due to a sharp decrease in muscle glycogen content. Costill et al (1985) observed a 40% reduction in muscle glycogen content following 4 weeks ‘detraining’. These values were virtually identical to a totally untrained individual. This may be due to a decrease in insulin sensitivity and GLUT 4 receptor activity (Costill, 1985).
V02Max and Endurance Performance
Following complete cessation of training, VO2max decreases in previously highly trained individuals after as little as 4 weeks, with the decrease in VO2max varying between 4 and 14% (Coyle, 1984). The decrease in VO2max during the first 3 weeks of detraining is due to a decrease in maximal cardiac output. Subsequent decreases in VO2max are due to decreased oxygen extraction, likely from a decrease in mitochondrial density (Houmard et al, 1989) Petibois and Déléris (2003), investigated changes in the metabolic response to an endurance exercise (18 rowing km at 75 % of maximal aerobic velocity) during detraining in ten rowers previously highly-trained. Maximal aerobic velocity (VO2 max) and the metabolic response to exercise were determined in the 1st, 24th, and 47th week (training), and in the 52nd, 76th, and 99th week (detraining). Short-term detraining (5 weeks) resulted in a lower adipose tissue triglyceride (TG) delivery during exercise (p = 0.029), but this one did not represent a direct metabolic limit to exercise since the liver TG delivery increased (p = 0.039), total fatty acid concentration remained unchanged. Long-term detraining (52 weeks) altered even more the metabolic response to exercise with a decreased total fatty acid concentration during exercise (week 99: 10.6 +/- 2.0 mmol/l; p = 0.022), which induced a higher glycolysis utilization.
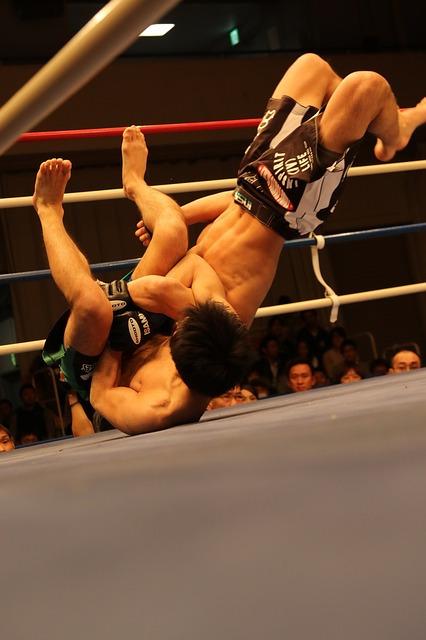
A haemolytic response to endurance exercise was observed through haptoglobin and transferrin concentration changes (weeks 47 vs. 99; p = 0.029 and 0.027, respectively), which resulted probably from higher red blood cell destruction. Endurance-trained athletes should avoid detraining periods over a few weeks since alterations of the metabolic adaptations to training may become rapidly chronic after such delays. (Petibois C & Deleris G, 2003).
Saltin et al (1968), examined the effect of 20 days of bed rest upon cardio respiratory endurance. On average the subjects experienced a 25% decrease in submaximal stroke volume, a 25% decrease in maximal cardiac output, and 27% decrease in maximal oxygen consumption. The reductions in cardiac output and V02Max appear to be related to the decrease in stroke volume, which in turn is probably due to a decrease in heart volume, total blood volume and ventricular contractibility.
The trained subjects experienced decreases in endurance compared to the untrained subjects; furthermore the untrained subjects regained their baseline fitness in 10 days, compared to 40 days for the trained subjects. This drop in conditioning may have implications for the ‘off season’ in many sports. Athletes should either maintain physical activity during the breaks from competition, or the pre season should begin over 40 days prior to the beginning of the competitive season. Giada et al (1998) studied 12 young and 12 older healthy sedentary males to examine the effects of training, and detraining on men of different age groups. Each subject underwent a maximal exercise test using a cycle-ergometer in order to measure maximum oxygen consumption and a echocardiography in order to assess left ventricle morphology and systolic function.
During the training period both groups of athletes showed higher values of maximum oxygen consumption, left ventricular wall thickness, end-diastolic diameter and volume, as well as left ventricular mass, than their control subjects. After the detraining period the wall thickness decreased only in young athletes, while left ventricular mass and end-diastolic diameter and volume reduced only in older athletes. It would appear from this study that the detraining effect may have many variables, one of which is age. However, further research using a greater number of subjects and control groups is warranted to provide a conclusive answer. Impairment of cardiovascular function following a few weeks of detraining is largely caused b a reduction in plasma volume, which in turn diminishes the plasma volume of the heart. Coyle et al (1986) observed a 9% decrease in blood volume and a 12% decrease in both stroke volume and plasma volume after two to four weeks of detraining following sustained periods of training, either running or cycling.
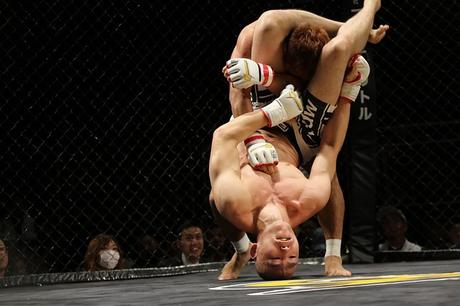
After the subjects were detrained, they were infused with dextrin solution to expand their blood volume until it exceeded their trained level. This improved cardiovascular function and V02max, but had little positive effect upon endurance performance. Reductions in cardio respiratory endurance appear to be much greater than reductions in strength and power over identical periods of detraining. Drinkwater and Horvarth (1972) studied seven female track athletes and again 3 months after training had ended. During the 3 month period, the athletes participated in typical physical activities for their age group, including physical education. At the end of the 3 months their V02Max had decreased by an average of 15.5%. Their new V02Max levels were similar to that of non-athletic girls the same age. Despite the rapid losses outlined in these studies, it would appear that a detraining effect only occurs if a person decreases their training volume by one thirds or more (Houmard et al, 1989), and/or their intensity falls below 70% Vo2Max.
Training for endurance or endurance sports differs from power and strength training in that it can be, and often is trained almost everyday (www.pponline.co.uk). Endurance can decrease in a matter of days after training has stopped. For example it has been shown that insulin sensitivity decreases to sedentary levels after just 10 days of rest (Gautier, 2004), leading to lower levels of muscle and liver glycogen. Athletes should include a period of reduced training intensity and frequency, but it is recommended that rest does not exceed 10 days and intensity does not fall below 70% V02Max.
Other Physiological Changes
Herd et al (1998) examined the effect of detraining upon postprandial lipeamia. Fourteen normolipidaemic, recreationally active young adults aged 18-31 years participated, in two self-selected groups: three men and five women (BMI 21·7-27·6 kg/m2) completed 13 weeks of running training, after which they refrained from exercise for 9 d; three men and three women (BMI 21·5-25·6 kg/m2) maintained their usual lifestyle. Oral fat tolerance tests were conducted at baseline and again 15 h, 60 h and 9 d after the runners’ last training session. In the absence of the acute effect of exercise, i.e. 60 h after the last training session, there was no effect of training on either postprandial lipaemia or on post-heparin lipo protein lipase activity. However, changes during 9 d of detraining in both these variables differed significantly between groups; after 2 d without exercise (60 h test), the runners’ lipaemic response was 37% higher than it was the morning after their last training session (15 h test; runners v. controls P , 0·05), with a reciprocal decrease in post-heparin LPL activity (P < 0:01). These findings suggest that improved fitness does not necessarily confer an effect on postprandial lipaemia above that attributable to a single session of exercise. Postprandial lipaemia is not elevated with training status and is therefore not influenced by detraining. It is however an acute response, and training must be regular and consistent in order to experience any benefits.
Retraining After Injury
Recovery of conditioning after a period of inactivity is affected by a person’s fitness level, length of training before the period of inactivity, and length of inactivity. Those who were fittest before inactivity suffer the greatest losses in fitness and also take longest to recover from inactivity. Two or three weeks of detraining have shown to cause the following decrements in highly trained subjects (Ross & Leveritt, 2001): Muscle oxidative enzymes decreased by 13 to 24% Performance time decreased by about 2 to 5% Vo2 max decreased by about 4% In a study conducted by Saltin et al (1968), 3 weeks of detraining resulted in significant losses in performance capacity. Following 15 days of retraining, only Vo2max had returned to its original trained level. Oxidative enzymes did not improve and although performance time showed some improvement, it still remained 2 to 5% below the trained time. This suggests that in elite athletes, the duration of retraining must exceed that of detraining in order to re-establish performance capacity to its original values.
Nutrition is an important consideration during times of inactivity. For example, a rugby player should decide whether or not to remain in positive energy balance in order to prevent further catabolism of muscle tissue, or should he try and remain as close as possible to a neutral energy balance in order to offset accumulation of fat tissue.
Van Baak (2004) showed that underfeeding by 250kcal per day over 6 weeks, resulted in the catabolism of 75% body fat and 25% fat free mass; underfeeding in addition to inactivity may result in massive in strength and power for the rugby player. Alpha Lipoic Acid mimics (to a certain extent) the anabolic effects of insulin (Eason et al, 2002). Loss of insulin resistance is on of the major causes of deconditioning during detraining, and therefore this supplement may be useful to injured athletes by preventing the unwanted accumulation of body fat, whilst keeping muscle lipid and glycogen levels high.
PEDs & Injury Recovery
Many athletes resort to illegal substance use in an attempt to offset the effects of inactivity or to accelerate the recovery process. Clenbuterol, a bronchodilator that used to be prescribed to asthmatics, is believed to poses anticatabolic properties, and is often used in the post cycle stages by steroid taking athletes, in order to avoid the catabolic effects of inhibited testosterone levels. Clinical research conducted by Montovani et al, 2001 has supported the theory that clenbuterol does possess some anti catabolic properties.
Anabolic steroids themselves have also been used by injured athletes in the past. Anabolic-androgenic steroids increase protein synthesis and therefore may mimic the effects of training, and repair tissue damage at an accelerated rate. Primabolin and stanozol are reputed as possessing the greatest anti catabolic properties, but stanozol, as a non-aromitising steroid (does not convert to oestrogen), is said to have a possible damaging effect upon joints by decreasing their fluid content. Conversely, aromatising steroids such as deca durabolin or deca nandrolone, are said to accelerate recovery from joint injuries by increasing the levels of synovial fluid within the joint capsule (MIMS, 2003).
TENS / NMES Electrical Stimulation for Injuries
Electrical Stimulation In one of the earliest published studies on the effects of high-intensity neuro-muscular electrical stimulation (NMES) on the maintenance of size and strength in immobilised muscles, researchers electrically stimulated the quadriceps and hamstrings on a daily basis for three weeks in the immobilised leg of an athlete wearing a lower-extremity cast as a result of Grade-II medial-collateral and anterior-cruciate ligament sprains in his knee (Pocari et al, 2003; Caggiano et al, 1994). On the day the cast was removed, the girth of the athlete’s thigh was increased, suggesting that muscle hypertrophy had occurred, instead of the usual cast-associated atrophy. In addition, single-leg, vertical-leap height was 92% as great in the immobilised leg following cast removal, compared with the uninjured leg, and the athlete was able to immediately return to competition.
The use of electrical stimulation to prevent muscle atrophy as a result of prolonged knee immobilisation following either injury or knee-ligament reconstructive surgery has been very intensely studied. Research (e.g. Pocari et al, 2003) has shown that electrical stimulation is effective in preventing decreases in muscle strength, muscle size, and even the oxygen-consumption capabilities of thigh muscles after knee immobilisation. In all but one of the studies in this area which have been published in scientific journals, electrical stimulation has been shown to be better in preventing negative changes in leg and knee-joint function, compared with no exercise, isometric exercise of the quadriceps-femoris muscles, and even isometric co-contractions of the quadriceps and hamstrings.
Studies suggested that these physiological and metabolic changes started after the 4-7th days of NMES application (Hudlicka et al, 1984) Type 2 fibrils prominently change to type 1 fibrils which are more rich for mitochondrial content, capillary density and oxidative enzyme capacity after electrical stimulation application, while the hypertrophy and hyperplasia in type 2 fibrils are more prominent after Isometric Exercise sessions (Cabric et al, 1998). This would suggest that isometric exercise may be of greater value to power athletes during injury, and electrical stimulation may be more important to endurance athletes.
Conclusion
This report has illustrated the way in which different aspects of fitness tend to deteriorate with inactivity or reduced training. It can be inferred from this that performance level in different sports will diminish at a rate determine by its specific demands.
However, over-training remains a problem with elite athletes, and it is generally agreed that an of-season period is required within which training intensity should be significantly reduced in order to maintain a high level of fitness without excessive strain upon the body (Smith, 2003, Roden, 2004).
Over-training can result in high levels of cortisol, free radicals and musculo-skeletal injuries. This will lead to a slow recovery from training sessions, susceptibility to illnesses and disease and ultimately, a decreased performance. Over-training symptoms include decreased appetite and body weight loss, muscle tenderness, head colds, nausea, sleep disturbances and elevated blood pressure (Willmore & Costill, 1999). This report concludes that injured athletes should attempt to train around injuries where possible, and take into consideration the possible influence that nutrition may have over their recovery.