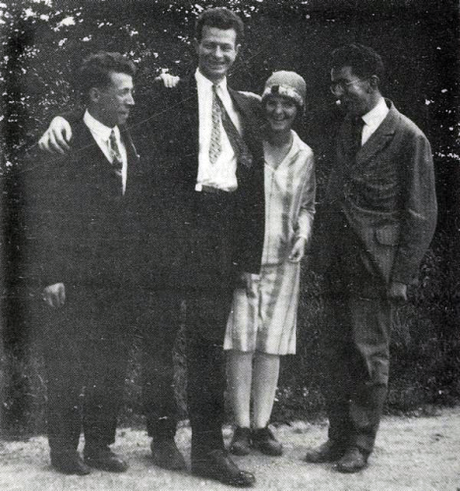
Linus and Ava Helen Pauling in Munich, with Walter Heitler (left) and Fritz London (right), 1927.
[An examination of Linus Pauling’s first paper on the nature of the chemical bond, published in April 1931. Part 2 of 2.]
In 1928 the German physicists Walter Heitler and Fritz London published a paper that appeared to have beaten Linus Pauling to the punch in its application of quantum mechanics to the theory of chemical bonding. As with Pauling, the duo was interested in Erwin Schrödinger’s wave function, and in their paper they applied it to the simplest bond: that formed by two hydrogen atoms. In so doing, Heitler and London did indeed become the first scientists to publish an application of this type.
The German colleagues also incorporated Werner Heisenberg’s ideas on exchange energy. Heisenberg had theorized that the electrons of two given atoms would find themselves attracted to the positively charged nuclei of their atomic pairs. As such, a chemical bond, according to this theory, consisted simply of two electrons jumping back and forth between two atoms, belonging simultaneously to both and to neither.
Heitler and London extended this idea, proposing that chemical bonds sourced their lengths and strengths from the amount of repulsion extant between two positively charged nuclei. The balance between the electrons’ attraction to these nuclei, coupled with the quantifiable repulsion existing between the two nuclei, ultimately served to create the bond.
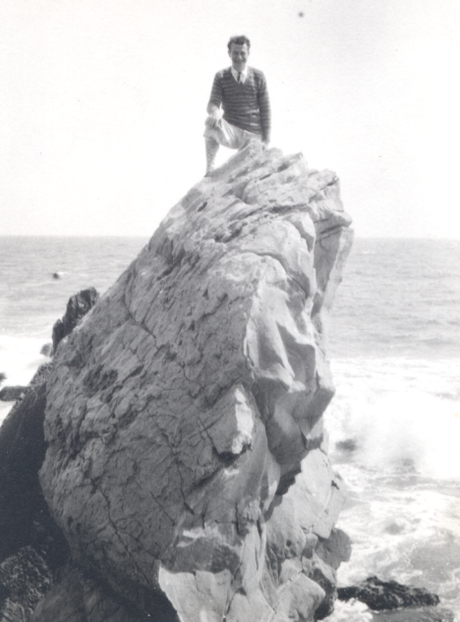
Pauling on the precipice of greatness, 1928.
Invigorated by the promise of competition, Pauling set to work applying Heitler and London’s theory to more complex molecules. In part to motivate himself, but also to ensure that he received recognition for his research, Pauling announced in the Proceedings of the National Academy of Sciences that he believed he could solve the tetrahedral binding of carbon using the ideas put forth by quantum mechanics.
This declaration piqued significant interest throughout the scientific community, striking a nerve for chemists and physicists alike, both groups of whom had been puzzling over this specific structure in different ways. On one hand, physicists believed that carbon should have a valence of two because, of its six electrons, four were located in two different subshells. Both sets of two would then be expected to pair off with each other, leaving only two electrons logically available for bonding. On the other hand, chemists found in the laboratory that carbon typically offered four electrons for bonding in nature. In essence, both theory and experiment indicated that neither party was completely right, but so too could neither point of view be completely wrong. Pauling believed that quantum mechanics could illuminate the paradox.
In addition to his theoretical study, Pauling’s extensive graduate training in x-ray crystallography strengthened both his interest in and his flair for atomic structure. By 1928, after a busy year of research, he had established five principles for determining the structure of complex covalent and ionic crystals, later dubbed “Pauling’s Rules.” He used these rules to predict models for particular molecular structures, and then worked backward from the theoretical model to develop a more concrete picture based on x-ray data. When a colleague remarked that this technique resembled the Greek stochastic method – an approach based largely on applied guess work – Pauling offered a correction, stating that
Agreement on a limited number of points cannot be accepted as verification of the hypothesis. In order for the stochastic method to be significant, the principles used in formulating the hypothesis must be restrictive enough to make the hypothesis itself essentially unique.
In other words, Pauling was relying on his knowledge of chemical principles to develop meticulous and educated hypotheses that he could go back and prove. And as he would hasten to add, he placed very little stock in luck or guesswork.
As work moved forward, Pauling added his rules to three others that had been established by G.N. Lewis – and then expanded and formalized by Heitler and London – concerning the electron pair bond. These rules set parameters for the circumstances in which an electron would be theoretically available to form a chemical bond.
Though equipped with a solid toolkit of his and others’ making, it ultimately took Pauling almost three years to solve the carbon tetrahedron, with his big breakthrough coming in December of 1930. Inspired by the work of MIT physicist John C. Slater, Pauling found a way to reduce the complexity of the radial wave function, a component of bond orbital theory the application of which had been giving him some trouble. With this solution in hand, the math required for solving further steps of the carbon puzzle became significantly more manageable.
Pauling’s subsequent equations led him to develop a model for the structure that consisted of four equal orbitals oriented at the angles of a tetrahedron. Using these equations, Pauling further discerned that the strength of the bonds within the structure increased in accordance with greater degrees of orbital overlap between two atoms. The overlap, Pauling found, produced more exchange energy and this in turn created a stronger bond.
Pauling sent his paper to the Journal of the American Chemical Society (JACS) in February 1931. In it, Pauling detailed three rules governing eigenfunctions that complemented G.N. Lewis’ rules about electron pairs. Pauling used this collection of guidelines to explain relative bond strength, finding that the strongest bonds occurred on the lowest energy level and where orbitals overlap. He also developed a complete theory of magnetic moments and ended the paper stressing the important role that quantum mechanics had played in his formulation of the rules and theories expressed in the work.
The paper, titled “The Nature of the Chemical Bond: Application of Results Obtained from the Quantum Mechanics and from a Theory of Paramagnetic Susceptibility to the Structure of Molecules,” was accepted and published in record time. The subject matter was so new and the ideas so fresh that Arthur Lamb, the editor of JACS at the time, had trouble finding a group qualified enough to review it. Even so, he scheduled the article for the April issue and in so doing published Pauling’s paper a mere six weeks after he had received it.
In 1926, whether he knew it or not, Linus Pauling embarked down a path toward the transformation of chemistry and the way that it would be studied for generations to come. The ideas that he began developing during this time gradually became the standard model for those studying chemistry while simultaneously launching Pauling to dizzying heights. His April 1931 paper, the first in a series of seven, also became the basis for his 1939 book, The Nature of the Chemical Bond, which was almost immediately recognized as a classic of twentieth-century scientific writing.
Largely on the strength of the April JACS article, Pauling also received the 1931 Langmuir Prize from the American Chemical Society, and used the money that came with the prestigious award to further his research. Now that he was interested in molecular structure, he saw it’s promise everywhere within a rapidly expanding research program. In fact, the chemical bond work of the late 1920s and early 1930s laid the foundation for his subsequent program of hemoglobin research, which in turn led to his sickle cell anemia discovery almost twenty years later. In hindsight, it is easy to see how Pauling could have looked back on the achievements of early 1931 as being “the best work I’ve ever done.”
Advertisements