R. B. Woodward et al., J. Am. Chem. Soc., 1952, 74, 4223 [PDF] (Full paper)
For preliminary communications see: JACS, 1951, 73, 3547 [PDF]; JACS, 1951, 73, 3548 [PDF]; JACS, 1951, 73, 4057 [PDF]; JACS, 1951, 73, 2403 [PDF]
Hundreds, if not thousands, of steroids have been characterised to date, isolated from a bewildering variety of organisms from across the animal, plant and fungal kingdoms. Their roles as hormones, drugs, and in cell membranes make them crucial to life as we know it, and are the reason that they’re one of the best studied classes of natural products. People have been interested in making steroids since the earliest days of total synthesis in the 1930s and 40s, and the field of steroid synthesis has made the careers of legendary chemists such as Russell Marker, George Rosenkranz, Arthur Birch and Carl Djerassi, as well as ensnaring and captivating many others. Indeed, some five Nobel Prizes have been awarded for steroid research, and the fruits of these labours have included many important drugs and much useful chemistry.
R. B. Woodward was also heavily involved in steroid chemistry during his early career, perhaps inspired by his PhD studies on ‘A Synthetic Attack on the Oestrone Problem’. As I wrote about in an earlier post, he also famously collaborated with Konrad Bloch to elucidate the details of steroid biosynthesis, work for which Bloch would receive the Nobel Prize in medicine the year before Woodward received his in chemistry. Woodward’s synthetic contributions to the field came in the form of a groundbreaking synthesis of methyl 3-keto-Δ4,9(11),16-etiocholatrienate, which he resolved and converted into a number of known compounds, achieving the formal total synthesis of some of the best known steroids.
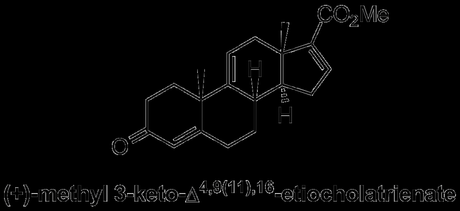
This flexible intermediate contained enough latent functionality (largely in the form of unsaturation in the carbon skeleton) to enable the interception of previously reported compounds that could be converted into cortisone, testosterone, progesterone and cholesterol, the archetypal members of four of the most important steroid families.
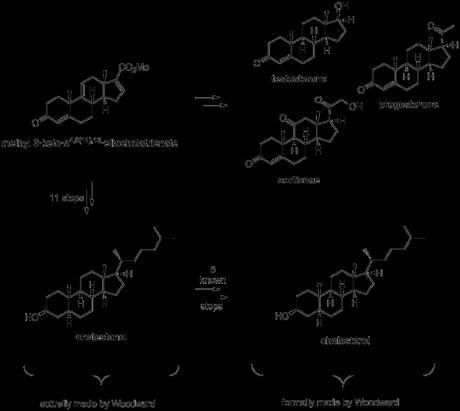
The route began with the seemly simple Diels-Alder reaction between butadiene and 4-methoxy-2,5-toluquinone, carried out on a typically Woodwardian scale involving the heating of 250 g of quinone in 500 mL diene in a sealed tube.[1] This was followed by a seemingly step that actually proved to be quite problematic: the base-mediated epimerisation of the cis decalin cycloadduct to the trans decalin required to proceed with the synthesis. Initial test reactions found that, unlike in simple, unsubstituted decalins and decalones, equilibration under basic conditions did not seem to favour the trans isomer to a great extent (if at all). Indeed, at first only about 40% trans compound was recovered from reactions that seemed to return large amounts of unchanged cis starting material. However, a breakthrough was made when it was discovered that the Diels-Alder adduct was readily soluble in aqueous sodium hydroxide solution and that isomerisation under these conditions sometimes—but not always—gave good yields of the trans product. The capricious nature of this reaction was eventually traced back to the existence of an interesting crystallisation equilibrium. In the more successful runs it appeared that adventitious initial crystallisation of a small amount of the trans isomer was serving as a seed to cause selective deposition of more of this isomer, pulling the equilibrium towards the desired product and driving the reaction to completion! Finally, the problem was solved when Woodward discovered that the addition of seeds of the trans isomer prior to acidification and isolation of the product led to high yielding, reproducible isomerisation every time. Next, the diketone was manipulated by reduction of both carbonyls, followed by enol ether hydrolysis and elimination of water. The remaining unwanted hydroxyl group was acetylated and then reductively removed by treatment with zinc in acetic anhydride.[2] According to Woodward's initial plan, the B-ring was to be constructed next, using Robinson’s eponymous annulation. First, however, the ketone α-position needed to be further activated, and this was accomplished by formylation with ethyl formate. Now, Michael addition through treatment with potassium tert-butoxide and ethyl vinyl ketone, followed by cyclisation and deformylation with aqueous sodium hydroxide gave the required tricyclic enone. Although the yield for this step was only moderate, according to the experimental section this step was carried out on scales of up to 2.5 kg, providing hundreds of grams of material with which to progress the synthesis.
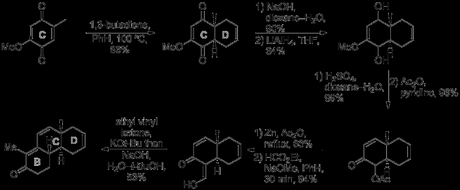
Resizing of the D-ring cyclohexene ring would ultimately require oxidative cleavage of the isolated double bond, and it was at this point that the group performed the first half of this transformation: dihydroxylation with osmium tetroxide.[3] Terrifyingly, this being a couple of decades before catalytic versions of this reaction were discovered, the reaction was actually performed with stoichiometric osmium tetroxide, and if that wasn’t bad enough, the osmate ester was actually isolated and studied! How’s this for a procedure:
“Osmium tetroxide (68.48 g.) dissolved in sodium-dried ether (750 cc.) was cooled in ice. The solution was added to the tricyclic ketone (XXXVI) (61.56 g.) dissolved in ice-cold ether (1.5 l.), with swirling. The mixture was set aside in the dark at room temperature for five to eight days, and the brown complex was then filtered off and washed with ether. It was dissolved in methylene dichloride (1 l.) and shaken mechanically with a solution of mannitol (350 g.) and potassium hydroxide (100 g.) in water (2.6 l.) until the organic layer became pale yellow (ca. one hour). The methylene chloride layer was separated, the aqueous layer was saturated with salt and extracted four times with chloroform. The combined organic solution were washed with salt solution, dried and evaporated at the water-pump. The residue (yield ca. 90%) crystallized when scratched. It was triturated with warm benzene (ca. 250 cc.), the mixture was cooled and the solid was filtered off. It weighed 49 g., and had m.p. 138–152º, Recrystallization from chloroform–benzene gave the isomer A (34.55 g., 49%), m.p. 152–156.5º (47–52% yields were obtained in other experiments). The analytical sample, obtained by crystallization from a large volume of benzene, formed silky needles, m.p. 157.5–158.5º. This isomer was used for the subsequent experiments.“
The diol thus obtained, whose stereochemistry was never determined, was protected as the corresponding acetonide, and selective hydrogenation of the disubstituted olefin was performed with palladium-on-stronium carbonate. It was now time for introduction of the final part of the core carbon skeleton – the A-ring. As the group’s plan called for selective alkylation of the α-position of the enone on the unsaturated side, it was first necessary to temporarily block the (more reactive) methylene at the α-position on the saturated side. This was done by a second α-formylation with ethyl formate, followed by conversion to the less reactive vinylogous amide by condensation with N-methylaniline. With the more reactive methylene safely protected, introduction of the final ring could now commence. This began with γ-deprotonation of the enone, followed by alkylation at the α-position of the dienolate with acrylonitrile. Hydrolysis of the resulting nitrile under basic conditions was then carried out, and heating the resulting acid in acetic anhydride with just ‘a trace’ of sodium acetate gave the rather unstable enol lactone.
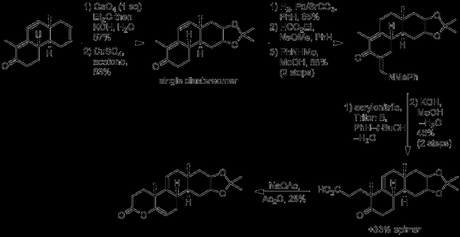
The enol lactone was opened with methyl magnesium bromide to give the corresponding methyl ketone, and this could finally be cyclised upon treatment with base to form the A-ring cyclohexenone! Now, all that remained to complete the target was resizing and manipulation of the D-ring. Thus, treatment with periodic acid achieved both acetonide deprotection along with oxidative cleavage of the resulting diol. The dialdehyde isolated from this reaction then underwent intramolecular condensation upon treatment with piperidine/acetic acid, producing predominantly the desired cyclopentenal. Finally, the aldehyde was converted to the corresponding acid and thence to the methyl ester to complete the synthesis of the racemictarget.[4][5] Resolution was carried out using the well-known, naturally occurring steroid glycoside digitonin.[6] The original paper is quite detailed with respect to this procedure, but here’s the short version: first, the enone was reduced the allylic alcohol with borohydride. This was then dissolved in 9:1 ethanol–water and treated with digitonin solution in the same solvent. This caused an enantioenriched mixture of the product and digitonin to precipitate out. Removal of the digitonin from this mixture, followed by a few recrystallisations and an oxidation (MPV) then gave the product essentially as a single enantiomer. Unfortunately, such a successful resolution came at the expense of yield, and only 12 mg of resolved product was obtained from 241 mg of racemic starting material!
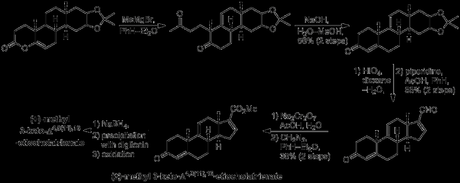
With a small amount of synthetic (+)-methyl 3-keto-Δ4,9(11),16-etiocholatrienate in hand, and helped by an injection of material derived from natural (+)-17α-hydroxycorticosterone 21-acetate (provided by Merck),[7] Woodward was now able to push on towards the key steroid targets cortisone, testosterone and progesterone. As you can see in the scheme below, the yields were a bit low, particularly in the hydrogenation steps. This was due to the mixtures of diastereomers obtained, which had to be painstakingly separated by precipitation with digitonin and/or multiple recrystallisations (1950s chromatography wasn't up to much). As a result of this, Woodward didn’t actually finish any of the target steroids himself, but rather achieved a formal synthesis via known transformations in each case.
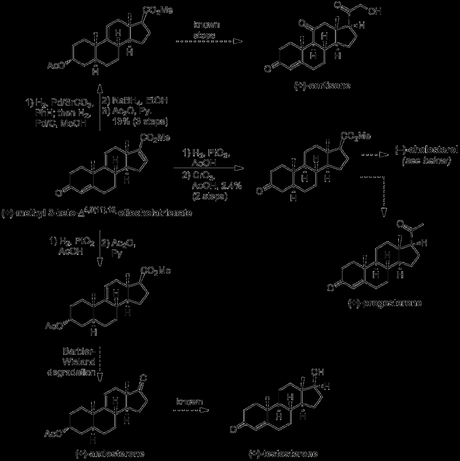
As far as cholesterol was concerned, a little extra work was required before its (formal) synthesis could be claimed. Work continued on from the saturated form of (+)-methyl 3-keto-Δ4,9(11),16-etiocholatrienate prepared above for the synthesis of progesterone, beginning with reduction of the ketone, hydrolysis of the ester and protection of the secondary alcohol as its acetate. Next, the carboxylic acid was converted to the corresponding methyl ketone, using an adapted literature procedure from a semi-synthesis of progesterone. This involved first making the acid chloride and then treating it with methyl cadmium (!), formed by reaction of methyl magnesium bromide—freshly prepared from neat methyl bromide (bp 3.5 ºC)—with cadmium chloride. I guess methyl cadmium is a pretty selective reagent as the reaction was conducted in refluxing benzene—conditions somewhat harsher than those usually required to react organometallics with acid chlorides—and the acetate protecting group remained untouched throughout! The last addition required to the molecule’s carbon skeleton was the introduction of the alkyl side chain. This was achieved by addition of a suitable Grignard reagent to the newly installed methyl ketone, followed by elimination of the resulting tertiary alcohol and reduction of the alkene formed. The rather low yield obtained for this sequence was mostly due again to problems separating the mixture of diastereomers obtained from the hydrogenation reaction, and this was again accomplished via diasteroselective precipitation with digitonin in the wake of unsuccessful chromatography. Finally, hydrolysis of the acetate gave cholestanol, which required only introduction of a double bond into the B-ring to complete the target, a simple feat using previously reported chemistry.
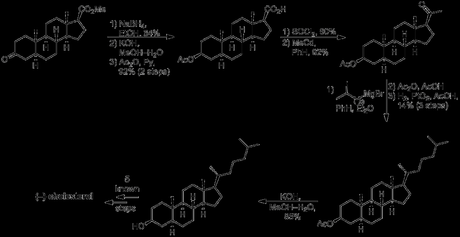
Although Woodward's steroid synthesis was long, low yielding, and more than a little impractical, it's important to remember the historical context: this was the first synthesis of any non-aromatic steroid, developed only a couple of years after the stereochemistry of the steroid skeleton was determined. The work was done with essentially no chromatography—excepting one or two partial separations on alumina pads—and structures were assigned using only IR, UV and CHN analysis, of compounds with up to 9 stereogenic centres in! To me, that sounds an awful lot like stumbling around in the dark, and yet Woodward's papers never read like that. There's always self assurance and aplomb; clear, easy to follow arguments and rationalisations; careful simplification of complex problems; fantastic footnotes; detailed literature precedent, and glorious experimental detail to back it all up! Although perhaps this work isn't as timeless as some of Woodward's classics like reserpine, which I reckon would still easily get into JACS today, this synthesis was a fantastic effort for its time. I'm not a steroid chemist, but I found this glimpse into the steroid era to be fascinating!
Etc.
1. I’m not sure how you ‘freshly distil’ or even measure butadiene as it boils at –4 ºC. The lowest boiling thing I’ve distilled (other than liquid ammonia) is acetaldehyde (bp 20 ºC), and that was very little fun at all.
2. The discussion in this paper is great, as is the length to which Woodward goes in order to explain the chemistry that he’s carrying out. The extensive use of footnotes containing interesting tangential information is a common theme in Woodward papers, as is mechanistic discussion (which was less common at the time), for example in this step, which is compared to the analogous reaction of the bromide:
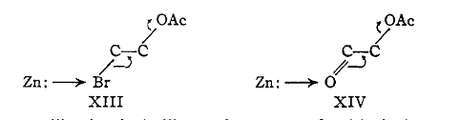
4. As this work pre-dates the Pinnick/Lindgren oxidation by more than two decades, that meant excess sodium dichromate/acetic acid (kinda like an early Corey-Schmidt). Mmmm mmm.
5. Would JACS accept proof of synthesis by melting point, appearance under the microscope and comparison of IR data these days? Maybe if the spectra looked like this:
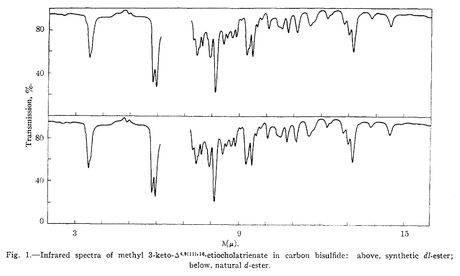
* A reaction from the days when men were men, and chemists in the pharmaceutical industry could invent—and use—reactions requiring chromium trioxide in pyridine. I actually did a couple of allylic oxidations under Sarett conditions during my PhD, and they worked a lot better than anything else I could think of. You see, typical chromium oxidation conditions (PCC, PDC…) are generally somewhat acidic, so Sarett’s conditions were developed to fill the niche for when you needed to apply chromium to really sensitive compounds. It’s not so common these days. If you plan on doing one, remember that dissolving CrO3 in pyridine is seriously exothermic, and a bit silly.
7. Not to be confused with the popular cardiac drug digoxin, which is also a steroid glycoside from the Digitalis (foxglove) family. Digitonin doesn’t have any cardiac effects (that Wikipedia or I know of), although it’s pretty useful in the biochemistry lab for solubilising lipids and membrane proteins as well as increasing the permeability of cell membranes.