Climate change is one ingredient of a cocktail of factors driving the ongoing destruction of pristine forests on Earth. We here highlight the main physiological challenges trees must face to deal with increasing drought and heat.
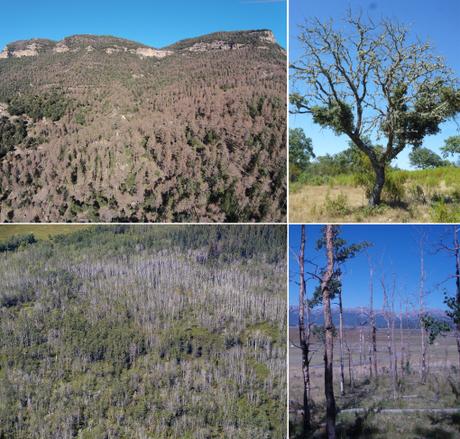
A common scene when we return from a long trip overseas is to find our indoor plants wilting if no one has watered them in our absence. But … what does a thirsty plant experience internally?
Like animals, plants have their own circulatory system and a kind of plant blood known as sap. Unlike the phloem (peripheral tissue underneath the bark of trunks and branches, and made up of arteries layered by live cells that transport sap laden with the products of photosynthesis, along with hormones and minerals — see videos here and here), the xylem is a network of conduits flanked by dead cells that transport water from the roots to the leaves through the core of the trunk of a tree (see animation here). They are like the pipes of a building within which small pressure differences make water move from a collective reservoir to every neighbours’ kitchen tap.
Water relations in tree physiology have been subject to a wealth of research in the last half a decade due to the ongoing die-off of trees in all continents in response to episodes of drought associated with temperature extremes, which are gradually becoming more frequent and lasting longer at a planetary scale (1).
Embolised trees
During a hot drought, trees must cope with a sequence of two major physiological challenges (2, 3, 4). More heat and less internal water increase sap tension within the xylem and force trees to close their stomata (5). Stomata are small holes scattered over the green parts of a plant through which gas and water exchanges take place. Closing stomata means that a tree is able to reduce water losses by transpiration by two to three orders of magnitude. However, this happens at the expense of halting photosynthesis, because the main photosynthetic substrate, carbon dioxide (CO2), uses the same path as water vapour to enter and leave the tissues of a tree.
If drought and heat persist, sap tension reaches a threshold leading to cavitation or formation of air bubbles (6). Those bubbles block the conduits of the xylem such that a severe cavitation will ultimately cause overall hydraulic failure. Under those conditions, the sap does not flow, many parts of the tree dry out gradually, structural tissues loose turgor and functionality, and their cells end up dying. Thus, the aerial photographs showing a leafy blanket of forest canopies profusely coloured with greys and yellows are in fact capturing a Dantesque situation: trees in photosynthetic arrest suffering from embolism (the plant counterpart of a blood clot leading to brain, heart or pulmonary infarction), which affects the peripheral parts of the trees in the first place (forest dieback).
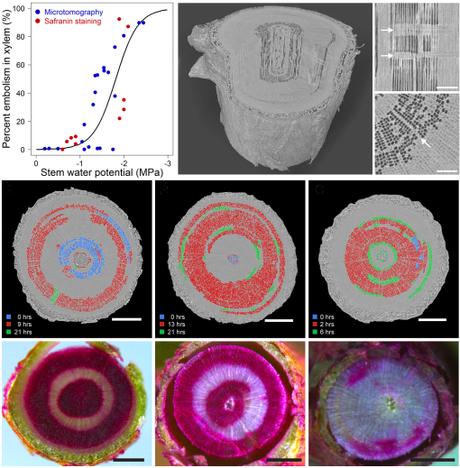
Lower: In black and white, microtomography of a trunk, and to the right (white scale = 100 microns) arrows point at xylem regions where embolism interrupts sap flow in vertical (upper) and horizontal (lower) planes. Colour photos (white and black scale = 500 microns) show three computer-colored trunk microtomographies of different saplings, each depicting a sequence of increasing embolism at different intervals in hours (hrs) from water privation (upper pics). Lower pics show, for three different saplings, the healthy areas of the xylem in pink since safranin does not stain dead tissue. Both methods corroborate that embolism compromises entire growth rings in sequoia saplings.
Perspectives
Global warming and extreme climates are altering the carbon cycle globally (see videos here, here, and here), where forests act as gigantic sinks of atmospheric carbon (7). Between 2001 and 2015, satellite data indicate that we have lost 314 million hectares of forest cover (> 5 times the Iberian Peninsula), supposedly as a result of agriculture, fire, logging and urbanisation (8).
However, much of that loss is in reality a climatic print that goes unnoticed by satellite imagery (9). Climate change is exerting, and will exert, rivalling effects on forests (10), and on the trophic networks (e.g., herbivores) that rely on them (11). Thus, more CO2 boosts photosynthesis, and explains up to 50% of the extended growth period detected in many forested areas of our planet — a phenomenon known as greening (12).
The problem is that greening requires healthy plants, which is utterly impossible during hot droughts and critically, in the months to years after those droughts. This is because trees cannot restore xylem function that has been previously damaged by severe embolism (2). In fact, when it comes to estimating forest-recovery time following embolism, greening plays a secondary role relative to the predominant effects of future droughts and peaks of hot and cold temperatures (13).
Exactly like in the animal kingdom, the plant kingdom (see documentary about botanic diversity) will also see losers and winners under anthropogenic climate change. For instance, massive mortality of trembling aspen (Populus tremuloides) in western North America, in response to severe droughts in 2000 to 2004, was driven by hydraulic collapse — not carbon stress (4). But each tree species is bound to have an idiosyncratic response to that pair of forcings.
Undoubtedly, those trees and those forests able to avoid embolism during periods of photosynthetic deficiency and high xylem pressure (5, 14) will be the fittest to withstand a planet currently undergoing extensive and increasing aridification. Every one of us can partly counteract it by planting trees every time we have the chance.
by Salvador Herrando-Pérez, David R. Vieites and Fernando Valladares
Note: a Spanish version of this blog has been published in the February 2019 issue of the magazine Quercus.
References
- Allen, CD et al. (2010). A global overview of drought and heat-induced tree mortality reveals emerging climate change risks for forests. Forest Ecology and Management 259: 660-684
- Choat, B et al. (2018). Triggers of tree mortality under drought. Nature 558: 531-539
- McDowell, N et al. (2008). Mechanisms of plant survival and mortality during drought: why do some plants survive while others succumb to drought? New Phytologist 178: 719-739
- Anderegg, WRL et al. (2012). The roles of hydraulic and carbon stress in a widespread climate-induced forest die-off. Proceedings of the National Academy of Sciences of the USA 109: 233-237
- Martin-St Paul, N et al. (2017). Plant resistance to drought depends on timely stomatal closure. Ecology Letters 20: 1437-144
- Brodribb, TJ & H Cochard (2009). Hydraulic failure defines the recovery and point of death in water-stressed conifers. Plant Physiology 149: 575-584
- Chuixiang, Yet al. (2015). Focus on extreme events and the carbon cycle. Environmental Research Letters 10: 070201
- Curtis, PG et al. (2018). Classifying drivers of global forest loss. Science 361: 1108-1111
- Klein, T & H Hartmann (2018). Climate change drives tree mortality. Science 362: 758-758
- Allen, CD et al. (2015).On underestimation of global vulnerability to tree mortality and forest die-off from hotter drought in the Anthropocene. Ecosphere 6: art129
- Carnicer, J et al. (2011). Widespread crown condition decline, food web disruption, and amplified tree mortality with increased climate change-type drought. Proceedings of the National Academy of Sciences of the USA 108: 1474-1478
- Zhu, Z et al. (2016). Greening of the Earth and its drivers. Nature Climate Change 6: 791-795
- Schwalm, CR et al. (2017). Global patterns of drought recovery. Nature 548: 202-205
- Skelton, RP et al. (2015). Predicting plant vulnerability to drought in biodiverse regions using functional traits. Proceedings of the National Academy of Sciences USA 112: 5744-5749
- Choat, B et al. (2016). Noninvasive measurement of vulnerability to drought-induced embolism by X-ray microtomography. Plant Physiology 170: 273-282