Tarantula Nebula - a star-forming region - as seen by the James Webb Space Telescope. NASA, ESA, CSA, STScI, Webb ERO Production Team, CC BY-SA " src="https://s.yimg.com/ny/api/res/1.2/cFa4vAHCW3CzQCbsak1olg-/YXBwaWQ9aGlnaGxhbmRlcjt3PTk2MDtoPTU1Mg-/https://media.zenfs.com/en/the_conversation_464/b6fe1e1c53c276f2d 160052ca86f9f63″ data-src="https://s.yimg.com/ny/api/res/1.2/cFa4vAHCW3CzQCbsak1olg-/YXBwaWQ9aGlnaGxhbmRlcjt3PTk2MDtoPTU1Mg-/https://media.zenfs.com/en/the_conversation_464/b6fe1e1c53c276f2d1 60052ca86f9f63″/>
Although our universe seems stable, having existed for a whopping 13.7 billion years, several experiments suggest that it is in danger - it is teetering on the edge of a very dangerous cliff. And it is all because of the instability of one fundamental particle: the Higgs boson.
In new research by me and my colleagues, recently accepted for publication in Physical Letters B, we show that some models of the early universe, which involve objects called low-mass primordial black holes, are likely wrong because they would have activated the Higgs boson by now and spelled the end of the universe.
The Higgs boson is responsible for the mass and interactions of all particles we know. That is because particle masses are a consequence of elementary particles interacting with a field, called the Higgs field. Because the Higgs boson exists, we know the field exists.
You can think of this field as a perfectly still water bath that we soak in. It has identical properties throughout the universe. This means that we observe the same masses and interactions in the cosmos. This uniformity has allowed us to observe and describe the same physics over several millennia (astronomers usually look back in time).
But the Higgs field is probably not in the lowest possible energy state that it could be in. That means that it could theoretically change its state, and drop to a lower energy state at a certain location. If that were to happen, however, it would drastically change the laws of physics.
Such a change would represent what physicists call a phase transition. This is what happens when water turns into vapor, creating bubbles in the process. A phase transition in the Higgs field would similarly create low-energy bubbles of space with completely different physics inside.
In such a bubble, the mass of electrons would suddenly change, and so would their interactions with other particles. Protons and neutrons-which make up the nucleus of an atom and are made of quarks-would suddenly change places. In fact, anyone who experiences such a change would probably not be able to report it.
Constant risk
Recent particle mass measurements from the Large Hadron Collider (LHC) at Cern suggest that such an event may be possible. But don't panic; this may not happen for a few trillion billion years after we retire. For this reason, the usual way of talking in particle physics departments is that the universe is not unstable, but rather 'metastable', because the end of the world is not coming anytime soon.
To form a bubble, the Higgs field needs a good reason. Because of quantum mechanics, the theory that governs the microcosm of atoms and particles, the energy of the Higgs field is always fluctuating. And it is statistically possible (though unlikely, which is why it takes so long) for the Higgs field to form a bubble from time to time.
However, the story is different with external sources of energy, such as strong gravitational fields or hot plasma (a form of matter made up of charged particles): the field can borrow this energy to more easily form bubbles.
While there is therefore no reason to expect the Higgs field to form numerous bubbles today, a major question in the context of cosmology is whether the extreme conditions shortly after the Big Bang could have created such bubbles.
However, when the universe was very hot, there was energy available to form Higgs bubbles, but thermal effects also stabilized the Higgs by changing its quantum properties. Therefore, this heat could not herald the end of the universe, which is probably why we are still here.
Primordial black holes
In our new study, we show that there is, however, one source of heat that would consistently drive such bubbles (without the stabilizing thermal effects seen in the early days after the Big Bang). These are primordial black holes, a type of black hole that formed in the early universe by the collapse of overly dense regions of spacetime. Unlike normal black holes, which form when stars collapse, primordial black holes can be tiny - as light as a gram.
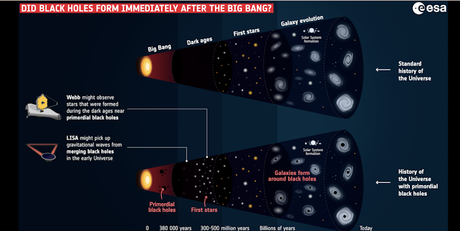
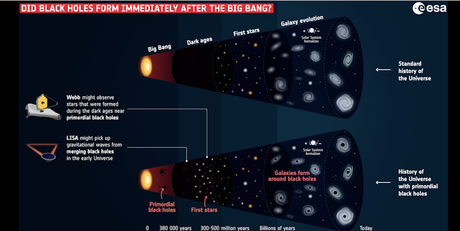
The existence of such low-mass black holes is a prediction of many theoretical models describing the evolution of the universe shortly after the Big Bang. These include some inflationary models, which suggest that the universe exploded in size after the Big Bang.
However, proving this existence comes with a major caveat: Stephen Hawking showed in the 1970s that, thanks to quantum mechanics, black holes slowly evaporate by emitting radiation through their event horizon (a point from which even light can no longer escape).
Hawking showed that black holes act as heat sources in the universe, with a temperature inversely proportional to their mass. This means that low-mass black holes are much hotter and evaporate faster than massive ones. In particular, if ancient black holes lighter than a few trillion grams (10 billion times less than the mass of the moon) formed in the early universe, as many models suggest, they would have evaporated by now.
In the presence of the Higgs field, such objects would behave like impurities in a soft drink. They would help the liquid form gas bubbles by adding to its energy through the effects of gravity (due to the mass of the black hole) and the ambient temperature (due to Hawking radiation).
When ancient black holes evaporate, they heat the universe locally. They would evolve at the center of hot spots that could be much hotter than the surrounding universe, but still colder than their typical Hawking temperature. What we showed, using a combination of analytical calculations and numerical simulations, is that, because of the existence of these hot spots, they would make the Higgs field bubble constantly.
But we're still here. That means it's highly unlikely that such objects ever existed. In fact, we should rule out all cosmological scenarios that predict their existence.
That is, of course, unless we find evidence of their former existence in ancient radiation or gravitational waves. If we do, that might be even more exciting. That would indicate that there is something we don't know about the Higgs boson; something that protects it from bubbling away in the presence of evaporating primordial black holes. These could, in fact, be brand new particles or forces.
Either way, it's clear that we still have much to discover about the universe, at the smallest and largest scales.
This article is republished from The Conversation under a Creative Commons license. Read the original article.
Lucien Heurtier is based at King's College London. Lucien Heurtier's work is partly supported by the UK Science and Technology Facilities Council (STFC) under GrantST/X000753/1