The primary scientific literature on climate change spawns hundreds of debates on an array of topics. When the technical debate among experts,and the obvious uncertainties, are taken up by the media, they are typically treated as any other topic, which ends up in some people not trusting science and others exploiting the ‘debate’ for their own interests.
Many media debates consist of one moderator and several speakers with two confronting views. When the topic under discussion affects our daily life (e.g., unemployment), the average spectator will often agree with one of the views. When the topic affects people (apparently) in a general fashion (e.g., climate change), the spectator might distrust or simply ignore both views. Thus, the media shapes public opinion such that people’s perception of the news becomes black, white, “I don’t believe it” or “it doesn’t exist”. Public debates on climate change are like a ‘contact sport’ (1), a team has to win in a contest where both parties alternate attack and defence. The participation of speakers without specialised expertise on climate change, especially if they represent short-term political and economic interests, instigates public mistrust and inhibition (2). This situation erodes the informative role that science and scientists must play in the creation of novel environmental policies aiming to improve the present and future wellbeing of our society (3, a Science paper unsurprisingly challenged by US administration’ bastion Fred Singer: 4).
–
Ocean acidification as example
Climate-change experts alert that, together with global warming, ocean acidification is “the other problem” of climate change (5). For species endowed with calcareous skeletons (e.g., algae, corals, molluscs, echinoderms), seawater acidity determines calcification rates (6). In the last decade, two iconic calcification experiments have casted completely opposite results for the photosynthetic algae Emiliana huxleyi (7, 8). Like all coccolithophores, the microscopic body of this planktonic species has a beautiful carapace of calcareous plates (coccoliths). Riebesell et al. (8) and Iglesias-Rodríguez et al. (7) cultivated E. huxleyi in aquaria at different concentrations of carbon dioxide CO2 (hence different acidity, see below). As populations grew in low- to high-CO2 water, Riebesell found a decline in calcification rates and more malformed skeletons, whereas Iglesias-Rodríguez encountered an increase in calcification rates and in the volume of the perfectly built skeletons.
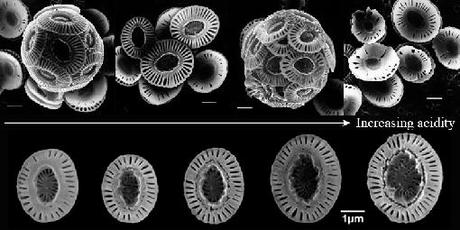
Coccolithophores of Emiliana huxleyi grown under increasing acidity (from left to right) in the lab. The upper row shows healthy individuals and cocoliths (left) versus malformed skeletons (right) (8). The lower row shows increase in the size of well-formed coccoliths (7). All white segments equal 1 micron (1 x 10-6 meters). Coccolithophores comprise ~ 300 species of unicellular algae, all of which are members of the marine phytoplancton. They live in the photic zone (allowing photosynthesis) where they form massive swarms that generate part of the air we breathe and fuel marine trophic webs, hence our fisheries. The synthesis of their skeletons represents one third of the calcium carbonate produced by oceans globally. Their fossils have been dated back to several hundred million years while the presence of alkenones allows the reconstruction of temperature changes over the geological history of our planet.
–
When the two research teams criticised each other in Science (9, 10), they provided contrasting arguments about the methods used to emulate ocean acidification in the lab. Out of such technical context, a methodological discussion (among others, i.e., 11) can turn into a discussion about whether ocean acidification exists or not – a perfect breeding ground for the deniers of climate change. For instance, the British newspaper The Independent (currently owned by a Russian businessman) flagged the study by Iglesias-Rodríguez in the article (23/04/2008): “Can seashells save the planet?”, asserting that coccolithophores “… are booming – and fighting climate change”.
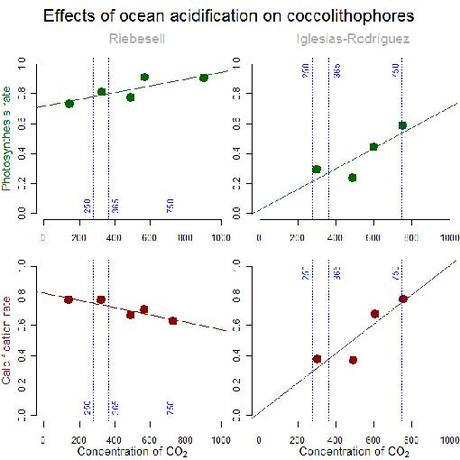
Calcification (carbon incorporation to coccoliths) and photosynthesis (carbon incorporation to soft tissues) rates according to different concentrations of carbon dioxide [CO2] in experimental populations of Emiliana huxleyi (7, 8). This apparently (15) cosmopolitan species of coccolithophore dominates in plankton communities of tropical, temperate and polar seas. With increasing [CO2], both studies report increasing photosynthetic activity, opposite calcification rates, and coccoliths with (8) and without malformations and larger coccospheres (7). [CO2] concentrations were created by adding acid/base (8) or by bubbling CO2 (7) in seawater aquaria, among other methodological differences (9, 10). Vertical lines represent preindustrial (250 ppmv), present (365 ppmv) and future (750 ppmv by the end of the century) [CO2].
–
There is no magic surrounding climate change
Increasing concentration of atmospheric CO2 has two major global consequences: warming and ocean acidification. It sounds almost magical, but it’s really a function of simple physical laws. CO2 is a greenhouse gas and, as such, returns to the Earth part of the sun’s heat our planet’s surface reflects. CO2 also dissolves in seawater at a rate proportional to its atmospheric concentration (Henry’s Law); once in seawater, it turns into carbonic acid. In turn, carbonic acid changes to bicarbonate and releases one proton (hydrogen atom with positive charge). The number of protons (known as pH) determines the acidity of a fluid. Therefore, the more CO2 in the atmosphere, the more CO2 in the ocean, the more free protons, the higher the acidity. This is how the concentration of marine CO2 has increased by some 40% over the last 200 years and is expected to triple the pre-industrial concentration by the end of the century under current rates of fossil-fuel burning (5). There is no evidence that such increased acidity has occurred in the oceans during last hundred million years (12).
Recent studies have revised the variety of impacts ocean acidification is exerting on marine species and on how we (humans) use them (5, 6, 13-16). These studies indicate that those impacts vary (varied and will vary) among regions, and that some species will benefit, but that most will suffer. The contrasting results by Iglesias-Rodríguez and Riebesell reflect that they used different strains of E. huxleyi, even different species (17); whereas field (18, 19) and laboratory (17, 20) studies of coccolithophores have found variable responses to acidification for different species, populations and genotypes. Apparently ‘conflicting’ results do not question the validity of single studies; rather, they emphasise the essence of ecology – that nature is mind-bogglingly complex. Scientists know the tools to handle and quantify the inherent variability in nature, but talking about climate change outside the technical context is bound to misinform.
–
–
Literature cited
- S. H. Schneider, Science as a contact sport: Inside the battle to save Earth’s climate. ( National Geographic, Washington, USA, 2009).
- N. Oreskes, E. M. Conway, Merchants of doubt: how a handful of scientists obscured the truth on issues from tobacco smoke to global warming. (Bloomsbury, New York, USA, 2010).
- P. Kitcher, Science 328, 1230 (Jun 4, 2010).
- S. F. Singer, Energy & Environment 21, 847 (Nov, 2010).
- S. C. Doney, V. J. Fabry, R. A. Feely, J. A. Kleypas, Annual Review of Marine Science 1, 169 (2009, 2009).
- J. Raven et al., “Raven, J. y otros autores (2005). Ocean acidification due to increasing atmospheric carbon dioxide” (The Royal Society, 2005).
- M. D. Iglesias-Rodríguez et al., Science (Washington), 336 (2008, 2008).
- U. Riebesell et al., Nature 407, 364 (Sep 21, 2000).
- M. D. Iglesias-Rodríguez et al., Science 322, (Dec 5, 2008).
- U. Riebesell et al., Science 322, (Dec 5, 2008).
- A. J. Andersson, F. T. Mackenzie, Biogeosciences 9, 893 (2012, 2012).
- K. Caldeira, M. E. Wickett, Nature 425, 365 (Sep 25, 2003).
- G. E. Hofmann et al., in Annual Review of Ecology, Evolution, and Systematics, Vol 41, D. J. Futuyma, H. B. Shafer, D. Simberloff, Eds. (2010), vol. 41, pp. 127-147.
- K. J. Kroeker, R. L. Kordas, R. N. Crim, G. G. Singh, Ecology Letters 13, 1419 (Nov, 2010).
- J. C. Orr et al., Nature 437, 681 (Sep 29, 2005).
- J. B. Ries, A. L. Cohen, D. C. McCorkle, Geology 37, 1131 (Dec, 2009).
- V. J. Fabry, Science 320, 1020 (May 23, 2008).
- L. Beaufort et al., Nature 476, 80 (Aug 4, 2011).
- H. E. K. Smith et al., Proceedings of the National Academy of Sciences of the United States of America 109, 8845 (Jun 5, 2012).
- A. Ridgwell et al., Biogeosciences 6, 2611 (2009, 2009).