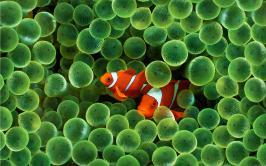
Clown fish couple (Amphiprion percula) among the tentacles of anemone Heteractis magnifica in Kimbe Bay (Papua New Guinea) – courtesy of Mark McCormick. Clownfish protect anemones from predators and parasites in exchange of shelter and food. The fish tolerates the host’s venom because its skin is protected by a mucus layer some 2-3× thicker than phylogenetically related species (12); clownfish fabricate the mucus themselves and seem to obtain anemone antigens through a period of acclimation (13), but whether protection is acquired or innate is still debated. Clownfish are highly social bony fish, forming groups with one reproductive pair (up to 11 cm in length each) and several smaller, non-reproductive males. Reproduction is protandrous (also known as sequential hermaphroditism), so larvae are born male and, as soon as the reproductive female dies, her widower becomes female and the largest of the subsidiary males becomes the alpha male. The IUCN lists clownfish, generically named ‘anemone fish’, as threatened by the pet-trade industry and habitat degradation, although surprisingly, only 1 species has been assessed (A. sandaracinos). The clown anemone fish A. ocellaris is the species that inspired Nemo in the 2003 Academy-Award fiction movie – contrary to the logical expectation that the Oscars Red Carpet would generate support for conservation on behalf of Hollywood, of the 1568 species represented in the movie, only 16 % of those evaluated are threatened (14).
Smell is like noise, the more scents we breathe in one sniff, the more difficult it is to distinguish them to the point of olfactory saturation. Experimental work with clownfish reveals that the increase in dissolved carbon dioxide in seawater, mimicking ocean acidification, alters olfactory physiology, with potential cascading effects on the demography of species.
Places such as a restaurant, a hospital or a library have a characteristic bouquet, and we can guess the emotional state of other people by their scents. Smell is critical between predators and prey of many species because both have evolved to detect each other without the aid of vision. At sea, the smell of predators dissolves in water during detection, attack, capture, and ingestion of prey, and many fishes use this information to assess the risk of ending up crunched by enemy teeth (1, 2). But predator-prey interactions can be modified by changes in the chemical composition of seawater and are therefore highly sensitive to ongoing ocean acidification (see global measuring network here). Experts regard ocean acidification as the ‘other CO2 problem’ of climate change (3) — just to emphasize that anthropogenic climate-change impacts terrestrial and aquatic ecosystems alike. Acidification occurs because the ocean absorbs CO2 at a rate proportional with the concentration of this gas in the atmosphere and, once dissolved, CO2 becomes carbonic acid (H2CO3), which in turn releases protons (H+) — in simple terms, pH is the concentration of protons (see video about ocean acidification):
Ocean-acidification (reversible) reaction (3)
CO2 + H2O « H2CO3 ⇔ HCO3– + H+ ⇔ CO32- + 2H+
Ocean acidification has doubled since the Industrial Revolution as a result of human-made CO2 emissions (4). Some call this phenomenon ‘hypercapnia’ (5) by analogy to the medical term for CO2 excess in blood. The fact is that acidification (or, hypercapnia) is triggering physiological, neurological, and ethological disorders in many aquatic species worldwide (4). Danielle Dixson has studied the problem in orange clownfish Amphiprion percula (5). Clownfish (Pomacentridae) comprise some 30 species, which dwell in the Indian and Pacific Oceans (see clownfish’s educational video and webpage). A. percula inhabits reefs in the Coral and Solomon Seas and the eastern coast of Papua New Guinea and the Great Barrier Reef in Australia. Females spawn thousands of eggs, leading to a planktonic larval stage that lives in the water column for about two weeks. When larvae reach adult morphology, they are recruited back to the reef habitat.
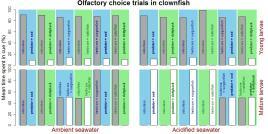
Experimental trials testing smell-based choices by clownfish Amphiprion percula in ambient against acidified seawater (6). Each panel (grey-white bar duet with green or blue background) is a 2-minute assay in which 15 larvae (individually) had to choose between two tunnels with contrasting smells including those of predatory (bommie cod Cephalopholis cyanostigma and brown dottyback Pseudochromis fuscus) or herbivore (mimic surgeonfish Acanthurus pyroferus and rabbitfish Siganus corallinus) fish [via aliquot cues of water from aquaria hosting both trophic guilds of fish], or odourless controls. Bar height indicates % mean time spent by clownfish in either tunnel per assay. Larvae for each assay were cultivated in captivity from 3 source adult pairs. At spawning, half of the eggs were incubated in ambient seawater (left panels), and the other half in ambient seawater acidified through CO2 bubbling (right panels). Every assay was replicated for newly hatched larvae (upper panels) versus 11-day larvae close to recruitment morphology (lower panels). Experiments demonstrate young larvae behave similarly in both types of water – always wary of other fish: friend or foe. Conversely, adult larvae eluded only predator-scented tunnels in ambient water, but in acidified water they could not differentiate predators from herbivores and were as untrustworthy of both.
Dixson analysed the behavior of young (right after hatching) and mature (just before recruitment) larvae in response to smell from natural predator versus herbivore fish. She used aquaria in which clownfish larvae had to decide to enter one of two tunnels injected with cues of predator or herbivore fish. Prior to the experiments, half of the larvae had been raised in ambient water (pH = 8.2), and the other half in acidified water at values predicted for this century in a climate-change scenario (pH = 7.8) (3).
In ambient water, young larvae preferred odour-free tunnels rather than tunnels carrying predator or herbivore cues (indicating smell discrimination of other species is not developed in newly born clownfish). In contrast, mature larvae only avoided tunnels scented with predators (indicating smell discrimination is already developed prior to recruitment, hence emerges between clownfish birth and adulthood). However, in acidified water, the choice of young larvae remained the same, but mature larvae lost their capacity of telling friend from foe.
Bicarbonate (HCO3–) is the compound resulting from the reaction of carbonic acid and seawater following CO2 dissolution and proton release (see formula above), and is a firm candidate to explain the sensorial disorders found by Dixson (5). Indeed, the pH of the blood stream of clownfish decreases in acidified water and affects the function of neurotransmitters, particularly GABA-A. This is the main inhibitor of the vertebrate brain, and goes on and off like a switch depending on the balance of chlorine and bicarbonate ions; unbalance drives nervous breakdown (like epilepsy in humans) and can alter the life history of species (6).
In the ocean, such impairment seems to affect not only olfaction, but also hearing, both of which control recognition among species as well as spatial orientation (7). So clownfish, and in general pomacentrid fish, can distinguish a reef by its smell (8) and sound (9), and this allows many larvae to return to their native reefs when they become adults (10). The aftermath of the effects of ocean acidification on multiple senses is that if they reduce survival or recruitment rates, they can compromise the (demographic) viability of marine wildlife (11), and ultimately, of our food stores.
—
by Salvador Herrando-Pérez & David R. Vieites
(with support of the British Ecological Society and the Spanish Ministry of Economy, Industry and Competitiveness)
References
- Brown, GE (2003) Learning about danger: chemical alarm cues and local risk assessment in prey fishes. Fish Fish 4: 227-234
- Wisenden, BD (2000) Olfactory assessment of predation risk in the aquatic environment. Phil Trans R Soc B 355: 1205-1208
- Doney, SC et al. (2009) Ocean acidification: the other CO2 problem. Annu Rev Mar Sci 1: 169-192
- McNeil, BI & Sasse TP (2016) Future ocean hypercapnia driven by anthropogenic amplification of the natural CO2 cycle. Nature 529: 383-386
- Dixson, DL et al. (2010) Ocean acidification disrupts the innate ability of fish to detect predator olfactory cues. Ecol Lett 13: 68-75
- Nilsson, GE et al. (2012) Near-future carbon dioxide levels alter fish behavior by interfering with neurotransmitter function. Nat Clim Change 2: 201-204
- Simpson, SD et al. (2011) Ocean acidification erodes crucial auditory behavior in a marine fish. Biol Lett 7: 917-920
- Gerlach, G et al. (2007) Smelling home can prevent dispersal of reef fish larvae. Proc Natl Acad Sci USA 104: 858-863
- Radford, CA et al. (2011) Juvenile coral reef fish use sound to locate habitats. Coral Reefs 30: 295-305
- Jones, GP et al. (2005) Coral reef fish larvae settle close to home. Curr Biol 15: 1314-1318
- Munday, PL et al. (2010) Replenishment of fish populations is threatened by ocean acidification. Proc Natl Acad Sci USA 107: 12930-12934
- Lubbock, R (1980) Why are clownfishes not stung by sea anemones? Proc R Soc Lond B 207: 35-61
- Elliott, JK et al. (1994) Do anemonefishes use molecular mimicry to avoid being stung by host anemones? J Exp Mar Biol Ecol 179: 99-113
- McClenachan, L et al. (2012) Extinction risk and bottlenecks in the conservation of charismatic marine species. Conserv Lett 5: 73-80