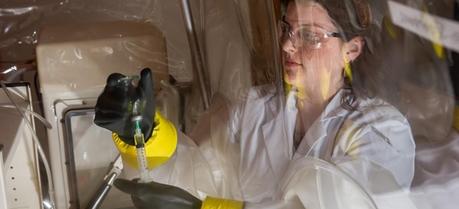
Methane hydrates are a potential energy source—only the already known methane hydrate reservoirs in the U.S. are expected to contain 6,700 trillion cubic feet of natural gas—more than the largest conventional natural gas fields. They, however, are also a potential source of global warming if massive amounts of methane were released during an earthquake or by rising ocean temperatures. A pair of methane-eating microbes may play a key role in this process.
Learning how these methane-munching organisms make a living in these extreme environments could provide clues about how the deep-sea environment might change in a warming world. Scientists already understood some details about the basic biochemistry of how these two organisms consume methane, but the details of the process have remained mysterious. The new study revealed that a rare trace metal—tungsten, also used as filaments in light bulbs—could be important in the breakdown of methane.
Natural gas hydrate or methane hydrate is a crystalline water-based solid, in which a large amount of methane is trapped within a crystal structure of water, forming a solid similar to ice. Originally thought to occur only in the outer regions of the Solar System where temperatures are low and water ice is common, significant deposits of methane clathrate have been found under sediments on the ocean floors of Earth. According to a study, around 6.4 trillion tonnes of methane is trapped in deposits of methane clathrate on the deep ocean floor; experts believe it is particularly abundant under the Arctic Ocean. This represents a potentially important future source of hydrocarbon fuel.“This is the first evidence for a microbial tungsten enzyme in low temperature ecosystems,” said Jennifer Glass, an assistant professor in the School of Earth and Atmospheric Sciences at the Georgia Institute of Technology.
The methane-eating organisms, which live in symbiosis, consume methane and excrete carbon dioxide.
“Essentially, they are eating it,” Glass said. “They are using some of the methane as a carbon source and most of it as an energy source.”
Phylogenetically speaking, one microbial partner belongs to the Bacteria, and the other is in the Archaea, representing two distinct domains of life. The archaea is named ANME, or anaerobic methanotrophic archaea, and the other is a sulfate-utilizing deltaproteobacteria. Together, the organisms form “beautiful bundles,” Glass said.
For a close-up view of the action on the sea floor, the research team used the underwater submersible robot Jason. The robot is an unmanned, remotely operated vehicle (ROV) and can stay underwater for days at a time. The research expedition in which Glass participated was Jason’s longest continuous underwater trip to date, at four consecutive days underwater.
The carbon dioxide excreted by the microbes reacts with minerals in the water to form calcium carbonate. As the researchers saw through Jason’s cameras, calcium carbonate has formed an exotic landscape on the ocean floor over hundreds of years.
“There are giant mountains on the seafloor of calcium carbonate,” Glass said. “They are gorgeous. It looks like a mountain landscape down there.”
While on the seafloor, Jason’s robotic arm collected samples of sediment. Back in the lab, researchers sequenced the genes and proteins in these samples. The collection of genes constitutes the meta-genome of the sediment, or the genes present in a particular environment, and likewise the proteins constitute a metaproteome. The research team discovered evidence that an enzyme used by microbes to “eat” methane may need tungsten to operate.
The enzyme (formylmethanofuran dehydrogenase) is the last in the pathway of converting methane to carbon dioxide, an essential step for methane oxidation.
Microorganisms in low temperature environments typically use molybdenum, which has similar chemical properties to tungsten but is usually much more available (tungsten is directly below molybdenum on the periodic table). Why these archaea appear to use tungsten is unknown. One guess is that tungsten may be in a form that is easier for the organisms to use in methane seeps, but that question will have to be answered in future experiments.
“We don’t know exactly why the organisms seem to be making a protein that binds the rare element tungsten instead of the more commonly used molybdenum,” Glass said.
Glass is currently writing a grant proposal to study a similar process in northern peatlands, which are large expanses of water and dead organic material. These peatlands, found in large expanses of high-latitude Canada, Europe and Russia, are significant sources of methane and that flux may increase with warming temperatures. Glass also plans to expand her research into oxygen-minimum zones, where large amounts of nitrous oxide are produced. Nitrous oxide is an important greenhouse gas and degrades the ozone layer.
“We want to understand on a gene level and on a chemical level, what’s going on in these processes, and then understand how this is going to change in the future with global warming and rising CO2,” Glass said.
Glass J.B., Yu H., Steele J.A., Dawson K.S., Sun S., Chourey K., Pan C., Hettich R.L., & Orphan V.J. (2013). Geochemical, metagenomic and metaproteomic insights into trace metal utilization by methane-oxidizing microbial consortia in sulfidic marine sediments. Environmental Microbiology PMID: 24148160