The word magic is not often used in the context of science. But in the early 1930s, scientists discovered that some atomic nuclei - the central part of atoms, which make up all matter - were more stable than others. These nuclei had specific numbers of protons or neutrons, or magic numbers, as physicist Eugene Wigner called them.
The race to find out what made these nuclei so stable began. Understanding these magic numbers can help scientists predict the properties of other nuclei, such as their mass or how long they are expected to live. This also allows scientists to predict which combinations of protons and neutrons can result in a nucleus.
The solution to the puzzle came from two directions at once in 1949. In the US, physicist Maria Goeppert Mayer published an explanation, while a group of scientists led by J. Hans D. Jensen in Germany found the same solution.
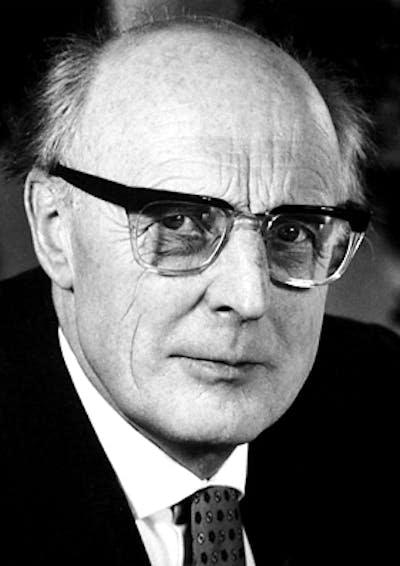
For their discovery, the two physicists each received a quarter of the 1963 Nobel Prize in Physics. We are two nuclear scientists whose work is based on the discoveries of Goeppert Mayer and Jensen 75 years ago. These magic numbers continue to play an important role in our research, but now we can study them in nuclei that live for only a fraction of a second.
Stability in the atom
The atom is a complex system of particles. It consists of a central nucleus made up of protons and neutrons, called nucleons, with electrons orbiting the nucleus.
Nobel Prize-winning physicist Niels Bohr described these electrons in the atom as existing in a shell structure. The electrons circulate around the nucleus in certain energy levels or orbits. These orbits have specific energies and each orbit can only hold a limited number of electrons.
Chemical reactions are the result of interactions between the electrons in two atoms. In Bohr's model, if an electron orbit is not yet filled, it is easier for the atoms to exchange or share those electrons and cause chemical reactions.
One class of elements, the noble gases, almost never reacts with other elements. In noble gases, the electrons occupy completely filled orbits, and as a result the atoms eagerly hold on to their electrons rather than sharing and undergoing a chemical reaction.
In the 1930s, scientists wondered whether protons and neutrons could also occupy orbits like electrons. But no one could prove this convincingly. For more than a decade, the scientific community was unable to describe the nucleus in terms of individual protons and neutrons. Scientists used a simpler picture, in which protons and neutrons were treated as a single system, like a drop of water.
Magic numbers
In 1949, Goeppert Mayer and Jensen developed the so-called shell model of the core. Protons and neutrons occupy certain orbits, analogous to electrons, but they also have a property called spin - similar to a spinning top. Goeppert Mayer and Jensen found that by combining the two properties in their calculations, they could reproduce the experimental observations.
Through some experiments, they discovered that nuclei with a certain magic number of neutrons or protons are unusually stable and retain their nucleons more than researchers previously expected, just as noble gases retain their electrons.
The magic numbers that scientists know are 2, 8, 20, 28, 50, 82 and 126. They are the same for both protons and neutrons. When a nucleus has a magic number of protons or neutrons, the orbit in question is filled and the nucleus is not very reactive, similar to the noble gases.
For example, the element tin has a magic number of protons. Tin always has 50 protons and the most common isotope has 70 neutrons. Isotopes are atoms of the same element that have different numbers of neutrons.
Nine other stable isotopes of tin may exist; this is the element with the largest number of stable isotopes. A stable isotope will never spontaneously change into another element, as is the case with radioactive isotopes.
Helium is the lightest 'doubly magical' nucleus with two protons and two neutrons. Both the number of neutrons and the number of protons are a magical number. The forces that hold the helium-4 nucleus together are so strong that it is impossible for another proton or neutron to attach to it. If you tried to add another proton or neutron, the resulting atom would immediately disintegrate.
On the other hand, the heaviest stable nucleus in existence, lead-208, is also a doubly magical nucleus. It has magic numbers of 82 protons and 126 neutrons.
Examples of magic numbers and stable nuclei exist everywhere - but scientists couldn't explain them without introducing the shell model.
Stable nuclei in nature
The shell structure in nuclei tells researchers about how elements are distributed on Earth and throughout the universe.
One of the most abundant elements on our planet and in the human body is oxygen, especially the isotope oxygen-16.
With eight protons and eight neutrons, oxygen-16 has an extremely stable nucleus. A nearby star produced the oxygen we find on Earth through nuclear reactions in its core, sometime before the solar system was formed.
Because oxygen nuclei are doubly magical, these nuclei in the star did not interact much with other nuclei. This left more oxygen to ultimately function as an essential ingredient for life on Earth.
In her Nobel lecture, Maria Goeppert Mayer talked about the work she did with physicist Edward Teller. The two had tried to describe how these elements formed in stars. In the 1930s it was impossible for them to explain why certain elements and isotopes are more abundant in stars than others. Later, she discovered that the increased numbers of nuclei corresponded to something in common: They all had a magic number of neutrons.
With the scale model and the explanation of magic numbers, the production of elements in stars became possible and was published in 1957.
Scientists today continue to use ideas from the nuclear shell model to explain new phenomena in nuclear science. A few accelerator facilities, such as the Facility for Rare Isotope Beams, where we work, aim to create more exotic nuclei to understand how their properties change compared to their stable counterparts.
At the Facility for Rare Isotope Beams, scientists produce new isotopes by accelerating stable isotopes to about half the speed of light and destroying them on a target. From the pieces we select the rarest and study their properties.
Perhaps the most profound modern discovery is the fact that the magic numbers turn into exotic nuclei like the type we are creating here. So 75 years after the original discovery, the race to discover the next magic number is still on.
This article is republished from The Conversation, an independent nonprofit organization providing facts and trusted analysis to help you understand our complex world. It was written by: Artemis Spyrou, Michigan State University and Sean Liddick, Michigan State University Read more: Artemis Spyrou receives funding from the US National Science Foundation. Sean Liddick receives funding from the Department of Energy, Office of Science and the Department of Energy, National Nuclear Security Administration.