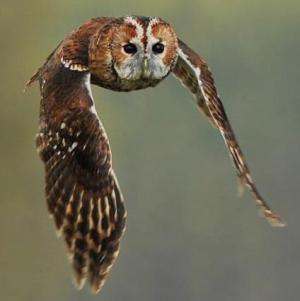
© Bill Doherty
Climate changes exert selective pressures on the reproduction and survival of species. A study of tawny owls from Finland finds that the proportion of two color morphs varies in response to the gradual decline of snowfall occurring in the boreal region.
Someone born in the tropics who travels to the Antarctic or the Himalaya can, of course, stand the cold (with a little engineering help from clothing, however). The physiology of our body is flexible enough to tolerate temperatures alien to those of our home. We can acclimate and, if we are healthy, we can virtually reside anywhere in the world.
However, modern climate change is steadily altering the thermal conditions of the native habitats of many species. Like us, some can live up to as much heat or cold as their genetic heritage permits, because each species can express a range of morphological, physiological, and behavioural variation (plasticity). Others can modify their genetic make-up, giving way to novel species-specific features or genotypes (evolution).
When genetic changes are speedy, that is, within a few generations, we are witnessing ‘microevolution’ — in contrast to ‘macroevolution’ across geological time scales as originally reported by Darwin and Wallace (1). To date, the detection of microevolution in response to modern climate change remains elusive, and many studies claiming so seem to lack the appropriate data to differentiate microevolution from phenotypic plasticity (i.e., the capacity of a single genotype to exhibit variable phenotypes in different environments) (2, 3).
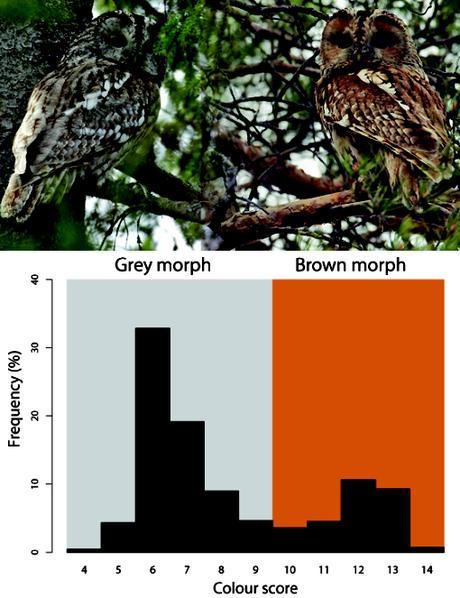
Colour morphs of tawny owls (Strix aluco) in the south of Finland. The histogram shows the frequency of plumages in a gradient from gray (left) to brown (right) in a sample of 3811 individuals collated from museum collections [3%], bird-ringing campaigns [84%] and direct observations by Karell et al. (4) [13%]. Colour scores are estimated by scoring from 4 (grey) to 14 (reddish-brown) four parts of the plumage (facial disc, back, breast, and overall appearance of wings, tail plus head). The dark colouration derives from pheomelanin, a pigment whose presence is independent of age and sex in this owl population (6). Photos courtesy of Dick Forsman.
Patrik Karell has documented outstanding evidence for microevolut
ion in tawny owls (Strix aluco) featuring two color morphs (brown versus grey) in a region of mixed forest and agricultural lands west of Helsinki (4). This region hosts one third of the Finnish population. Tawny owls are monogamous predatory birds (weight ~ 500 grams, wingspan ~ 1 metre, longevity ~ 4 years) — see here for a hunting scene. It is a ‘Least Concern’ species for the IUCN, with an European population of up to one million pairs, and a global distribution spanning from the North of Africa to the West of Asia.
Between 1980 and 2008, Karell ringed 491 individuals, totalling more than one thousand captures and re-captures, which allowed estimates of survival and colour-plumage inheritance rates. He found that almost 80% of the color variation across generations had a genetic basis (i.e., was heritable and independent of phenotypic plasticity), and appeared to be governed by a single gene whereby the ‘brown’ allele dominates the ‘grey’ one.
In demographic terms, brown morphs in their reproductive age increased from 24 (12% of the population) to 348 (42%) individuals in Finland over the study period, and such increase was of similar magnitude in the study region and was not due to the arrival of foreign individuals from elsewhere (according to capture-mark-recapture data).
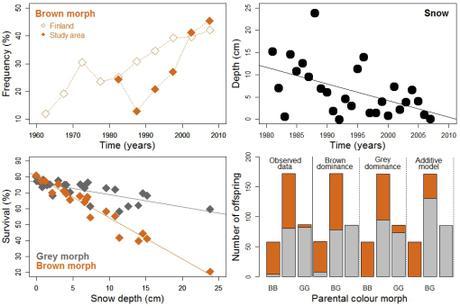
Demographic and genetic aspects of tawny owls (Strix aluco) in Finland (4). Upper panels show the proportion of brown morphs (relative to the total number of brown and gray morphs) ringed from 1960 to 2010 [left], and the depth of the snow layer in the study area between 1981 and 2008 [right]. The lower-left panel shows the correlation between snow depth and owl survival for both morphs. The three graphs support higher abundance and survival for brown than for gray individuals as snow depth diminishes. The lower-right panel presents data for 318 offspring re-captured as adults and for which the colouration of their parents was known (BB = both brown, GG = both grey, BG = one brown + one grey): bars furthest to the left show observed frequencies of the two morphs, and the remaining bars represent theoretical (i.e., model-based) frequencies assuming that plumage color is determined by one single gene (with brown or gray as dominant, middle bars), or by the additive effect of multiple genes (bars furthest to the right). The brown-dominant, single-gene model predicts theoretical frequencies closest to observed frequencies.
Most interestingly, the study reveals that owl survival rates (i) decline as temperatures drop, and (ii) depend on how much snow falls, particularly for brown individuals. Thus, given the attenuation of snowfall in the south of Finland in the last five decades (5), the survival of the brown morph triples with each centimeter of snow-layer deficit over time, and has resulted in similar numbers of brown and gray individuals by the end of 2010s.
The increased frequency of mild winters in Finland from the middle of the 20th Century is favouring browner tawny owls, despite the observation that, on average, brown morphs produce about half fewer offspring than gray morphs (4, 6). Such demographic paradox could be caused by the brown plumage enhancing crypsis against predators under snow-poor conditions. Additionally, brown owls have been observed to exploit food resources more efficiently than gray owls (7), indicating potential genetic covariation of melanisation and other life-history traits, e.g., the capacity to adapt to varying food supplies and parasite loads (7, 8).
Overall, the integration of future microevolutionary studies for multiple species with contrasting life histories will be crucial to distinguish those traits and genes that are variable enough to keep up with the pace of modern climate change – and so prevent population and species extinctions (9).
—
by Salvador Herrando-Pérez & David R. Vieites
Museo Nacional de Ciencias Naturales-CSIC. @vieites, @hp_salva. Funded by the British Ecological Society and the Spanish Ministry of Economy, Industry and Competitiveness
—
References
- Reznick, D. N. & Ricklefs, R. E. (2009). Darwin’s bridge between microevolution and macroevolution. Nature, 457: 837-842
- Gienapp, P. et al. (2008). Climate change and evolution: disentangling environmental and genetic responses. Molecular Ecology, 17: 167-178
- Merilä, J. (2012). Evolution in response to climate change: in pursuit of the missing evidence. Bioessays, 34: 811-818
- Karell, P. et al. (2011). Climate change drives microevolution in a wild bird. Nature Communications, 2: 208
- Irannezhad, M. et al. (2016). Wintertime climate factors controlling snow resource decline in Finland. International Journal of Climatology, 36: 110-131
- Brommer, J. E. et al. (2005). The color of fitness: Plumage coloration and lifetime reproductive success in the tawny owl. Proceedings of the Royal Society B, 272: 935-940
- Piault, R. et al. (2009). Pheomelanin-based coloration and the ability to cope with variation in food supply and parasitism. The American Naturalist, 174: 548-556
- Ducrest, A. L. et al. (2008). Pleiotropy in the melanocortin system, coloration and behavioural syndromes. Trends in Ecology and Evolution, 23: 502-510
- Reusch, T. B. & Wood, T. E. (2007). Molecular ecology of global change. Molecular Ecology, 16: 3973-3992