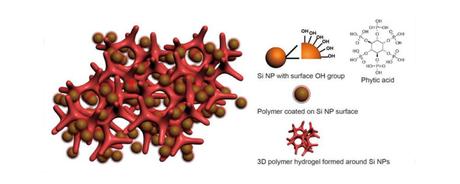
This is an illustration of a new battery electrode made from a composite of hydrogel and silicon nanoparticles (Si NP). Each Si NP is encapsulated in a conductive polymer surface coating and connected to a three-dimensional hydrogel framework. (Credit: Yi Cui, Stanford University)
The most commercially popular negative electrode material used in lithium-ion batteries is graphite. While silicone could be a much more efficient choice, all silicon-based designs have a major flaw—these structures tend to crack or break easily when they are used repeatedly. Scientists from the University of Maryland have recently proposed to solve this problem by growing special silicon nanostructurs. Now, researchers at the Stanford University have significantly improved the performance of lithium-ion batteries by creating novel electrodes made of silicon and conducting polymer hydrogel, a spongy substance similar to the material used in soft contact lenses and other household products.
Writing in the June 4 edition of the journal Nature Communications, the scientists describe a new technique for producing low-cost, silicon-based batteries with potential applications for a wide range of electrical devices.
“Developing rechargeable lithium-ion batteries with high energy density and long cycle life is of critical importance to address the ever-increasing energy storage needs for portable electronics, electric vehicles and other technologies,” said study co-author Zhenan Bao, professor of chemical engineering at Stanford.
To find a practical, inexpensive material that increases the storage capacity of lithium-ion batteries, Bao and her Stanford colleagues turned to silicon—an abundant, environmentally benign element with promising electronic properties.
“We’ve been trying to develop silicon-based electrodes for high-capacity lithium-ion batteries for several years,” said study co-author Yi Cui, associate professor of materials science and engineering at Stanford. “Silicon has 10 times the charge storage capacity of carbon, the conventional material used in lithium-ion electrodes. The problem is that silicon expands and breaks.”
Studies have shown that silicon particles can undergo a 400 percent volume expansion when combined with lithium. When the battery is charged or discharged, the bloated particles tend to fracture and lose electrical contact. To overcome these technical constraints, the Stanford team used a fabrication technique called in situ synthesis polymerization that coats the silicon nanoparticles within the conducting hydrogel.
A new way
This technique allowed the scientists to create a stable lithium-ion battery that retained a high storage capacity through 5,000 cycles of charging and discharging. “We attribute the exceptional electrochemical stability of the battery to the unique nanoscale architecture of the silicon-composite electrode,” Bao said.
Using a scanning electron microscope, the scientists discovered that the porous hydrogel matrix is riddled with empty spaces that allow the silicon nanoparticles to expand when lithium is inserted. This matrix also forms a three-dimensional network that creates an electronically conducting pathway during charging and discharging.
“It turns out that hydrogel has binding sites that latch onto silicon particles really well and at the same time provide channels for the fast transport of electrons and lithium ions,” explained Cui, a principal investigator with the Stanford Institute for Materials and Energy Sciences at the SLAC National Accelerator Laboratory. “That makes a very powerful combination.”
A simple mixture of hydrogel and silicon proved far less effective than the in situ synthesis polymerization technique. “Making the hydrogel first and then mixing it with the silicon particles did not work well,” Bao said. “It required an additional step that actually reduced the battery’s performance. With our technique, each silicon nanoparticle is encapsulated within a conductive polymer surface coating and is connected to the hydrogel framework. That improves the battery’s overall stability.”
Addressing the fire issue
Hydrogel primarily consists of water, which can cause lithium-ion batteries to ignite—a potential problem that the research team had to address. “We utilized the three-dimensional network property of the hydrogel in the electrode, but in the final production phase, the water was removed,” Bao said. “You don’t want water inside a lithium-ion battery.”
Although a number of technical issues remain, Cui is optimistic about potential commercial applications of the new technique to create electrodes made of silicon and other materials.
“The electrode fabrication process used in the study is compatible with existing battery manufacturing technology,” he said. “Silicon and hydrogel are also inexpensive and widely available. These factors could allow high-performance silicon-composite electrodes to be scaled up for manufacturing the next generation of lithium-ion batteries. It’s a very simple approach that’s led to a very powerful result.”
Former Stanford postdoctoral scholars Hui Wu, now a faculty member at Tsinghua University-Beijing, and Guihua Yu, now a faculty member at the University of Texas-Austin, are co-lead authors of the study. Other authors are Stanford visiting scholar Lijia Pan and graduate students Nan Liu and Matthew McDowell.
By Mark Shwartz
Wu, H., Yu, G., Pan, L., Liu, N., McDowell, M., Bao, Z., & Cui, Y. (2013). Stable Li-ion battery anodes by in-situ polymerization of conducting hydrogel to conformally coat silicon nanoparticles Nature Communications, 4 DOI: 10.1038/ncomms2941