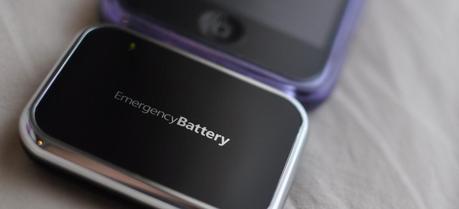
Scientists at McCormick School of Engineering and Applied Science have combined two traditional theories in materials science that can explain how the charge dictates the structure of the material. This opens the door for many applications, including a new class of batteries.
A lithium-based battery has a major disadvantage: it’s highly flammable, and when it overheats, it can burst into flames. For years, scientists have searched for safer battery materials that still have the same advantages as lithium. While plastics (or polymers) seemed like an obvious choice, researchers never fully understood how the material would change when an ion charge was introduced.
SEE ALSO: Technology Prevents Spontaneous Combustion in Li-Ion Batteries
“There is a huge effort to go beyond lithium in a flammable solvent,” says Monica Olvera de la Cruz, Lawyer Taylor Professor of Materials Science and Engineering and senior author of the paper. “People have been looking at alternatives that are not explosive, like plastics. But they didn’t know how to compute what happens when you put in a charge.”
The team looked at plastics known as block copolymers (BCPs) that are two types of polymers stuck together. They are a leading material for use as ion conductors because they self-assemble into nanostructures that both enable ion charge transport and maintain structural integrity. BCPs innately have nano-channels through which the ion can travel, but the charges themselves manipulate the shape of the channels. To use the material in batteries, researchers must find a way to control the shape of the nano-channels, so that the charge moves well.
“If you can optimize the ability of the charge to move through the system, then you can optimize the power that actually comes out of the battery,” says Charles Sing, a postdoctoral fellow in Olvera de la Cruz’s lab and first author of the paper.
The problem lies in the structure of the material. BCPs are very long chains of molecules. When they are stretched out, they extend over distances much greater than the typical size of the ion charges. However, the charges still have a strong effect on the nano-channels despite being much smaller. To properly understand the dynamics of BCPs, different theories are needed for the different length scales.
To understand how the ion charge changes the structure of the BCPs’ nano-channels, Sing and Jos Zwanikken, a research assistant professor in the same lab, combined two traditional theories: the Self-Consistent Field Theory and Liquid State Theory. Self-Consistent Field Theory describes how long molecules behave.
“Liquid State Theory, on the other hand, describes how charges operate on very local, atomic levels,” Zwanikken says.
While these two theories have been studied, in-depth, for decades, no one has previously put them together. When combined, they provide a new way of looking at the nano-channel systems. The electrical charge, known as an ion, is associated with an oppositely charged molecule, known as a counter-ion, which is also present in the nano-channel. Together, these ions and counter-ions are highly attracted to each other and form a salt. These salts cluster into miniature crystals, which exert a force on the nano-channels, changing their structure.
Olvera de la Cruz and her group found that these two effects balance one another—the salts want to form mini-crystals, which forces the nano-channel to deform. This understanding makes it possible to predict and even design a “highway system” through which the ions are transported, maximizing the power of the battery.
The team hopes their finding will guide experimentalists as they test materials. It will give researchers more information about the physical concepts underlying BCP systems.
Olvera de la Cruz says, “We have provided the tools to understand these systems by including ionic-length scale effects into the polymer mesoscale morphology.”
Sing, C., Zwanikken, J., & Olvera de la Cruz, M. (2014). Electrostatic control of block copolymer morphology Nature Materials DOI: 10.1038/nmat4001