I originally started writing this post before Christmas but then lost the near-completed version with the death of my laptop. However, I recently found some old backups and decided to finish it up and put it online for your enjoyment. Have fun!---BRSM.
Total Synthesis of (+)-Crotogoudin
S. Breitler and E. M. Carreira*, Angew. Chem., Int. Ed., 2013, 52, 11168; [PDF][SI][GROUP]
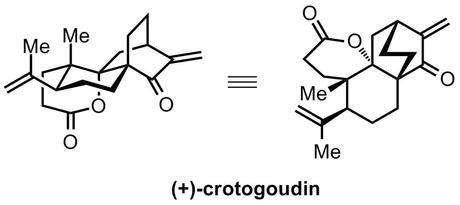
As the name implies, crotogoudin is another natural product from the goldmine of bioactive compounds that is the Croton genus of flowering trees. Seeds of these plants have been used for hundreds of years to produce the famous croton oil, a violent emetic and purgative used in early medicine across the globe before anyone realised just how bad it really was. Now, the notorious extract is mostly used as a source of various natural products, a reproducible way of inducing pain and/or irritation in animal experiments and a case in point that things described as 100% natural can still be extremely bad for you. It also serves as the major source of the important natural product phorbol, and gave its name to crotonic and tiglic acid (and thus crotonaldehyde).[1] Along with a number of other natural products isolated from this genus, crotogoudin displays promising cytotoxic activity, which, coupled with the rare 3,4-seco atisane skeleton, was probably one of the reasons that the Carreira group recently embarked on its total synthesis.
The group envisaged the use of a radical cyclisation as the key step to form the final ring in the natural product, the required radical generated from the reductive opening of a cyclopropyl ester as shown below. The precursor to this reaction could be prepared from the simpler chiral β-hydroxyketone, a building block that could be easily obtained in enantiopure form by enzymatic desymmetrisation of the corresponding meso-diketone. Although the use of enzymes to produce chiral starting materials is by no means a recent development, it remains a fairly uncommon sight in total synthesis,[2] possibly because such reactions are limited to the preparation of a relatively small number of simple building blocks—desymmetrisation works best for diols, diesters and diketones, for example—and it’s not always easy to design efficient routes around these starting materials. Additionally, large amounts of substitution around these motifs is often not well tolerated as the enzyme’s active site is just not able to fit such unnatural substrates in; unlike new catalysts promising implausible substrate scope and generality, enzymes usually have evolved to be very fussy about what molecules they'll accept. Of course, with the advent of synthetic biology, it’s becoming increasingly possible to retool enzymes for our own purposes, and I think that this approach become a lot more popular over the next decade (especially in industry, where it’s more reasonable to spend huge sums of money to find a way of optimising a single step to perfection).[3] Anyway, that’s probably the subject of another blog post, and—at least in the case of this synthesis—Nature's capabilities are adequate, and the reaction is a good fit for the route!
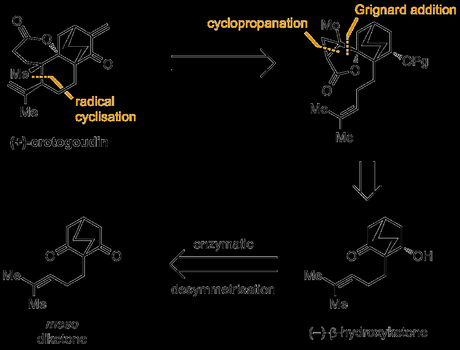
The route began with a one-pot Michael addition–Aldol reaction between a β-ketoester and an enal, and this was swiftly followed by a Kraptcho-type decarboxylation to excise the unwanted ester.[4] The benzyl protecting group was then removed under Birch conditions, which also resulted in 1,4 and 1,2 reduction of the enone (all in just 8 minutes!).[5] A double oxidation of the primary and secondary alcohols was then carried out under Swern conditions, and the resulting ketoaldehyde was then treated with dilute hydrochloric acid to effect an intramolecular Aldol reaction and give the racemic β-hydroxyketone. In order to carry out the required desymmetrisation this had to be reoxidised, and the group accomplished this in good yield using the Dess–Martin periodinane. Conveniently, no purification was required between the Birch and Dess–Martin steps, allowing material to be easily advanced through this part of the synthetic sequence. Finally, with the meso-diketone in hand, treatment with baker’s yeast in aqueous ethanol returned the previous β-hydroxyketone in good yield, and with >99% ee.
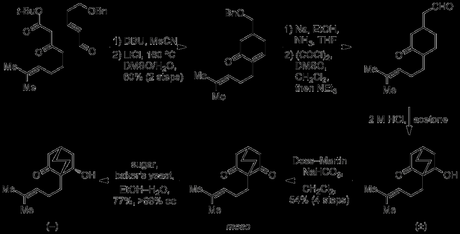
Next, the group needed to construct the lactone ring required for the annulation cascade, and this required the addition of 2-propenylmagnesium bromide to the ketone. Initially, the group capped the free alcohol with a TBS group prior to attempting the reaction, but curiously the protected substrate proved to be entirely unreceptive to any nucleophiles tried. In light of this failure they then tried performing the addition on the unprotected β-hydroxyketone, and did obtain some desired product, albeit in low yield. As addition to the ketone was clearly not a particularly rapid or facile process, the group reasoned that competing enolisation, with the Grignard acting as a base rather than a nucleophile, could be the problem. Sure enough, when the group instead used the much less basic organolanthanum reagent as the nucleophile then they were finally able to get their desired product in good yield.
With the organometallic addition complete, the secondary alcohol was selectively protected, leaving the newly formed tertiary alcohol free. Next, the group needed to find conditions that would allow them to carry out selective cyclopropanation of the 1,1-disubstituted over the trisubstituted olefin in the side chain. Fortunately, they found that rhodium-catalysed cyclopropanation with a phenyliodonium malonate as the carbene source gave a reasonable yield of the desired product, and with moderate selectivity for the desired diastereomer.
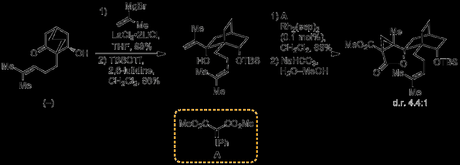
With the radical cyclisation precursor in hand, the group found that treatment with samarium diiodide did effect the crucial ketyl formation–ring expansion–radical cyclisation sequence, but the desired tetracycle was only obtained in 10% yield, along with other products where the side chain double bond had been reduced, resulting in an unusable isopropyl group. They hypothesised that these by-products arose from a second reduction of the tertiary radical formed in the cyclisation step, followed by protonation of the resulting tertiary anion. Thus, in order to prevent this undesirable over-reduction, they decided to install a leaving group at the allyl position that could be eliminated once the anion had formed, hopefully before protonation could occur. This was done by allylic oxidation with selenium dioxide, followed by protection of the resulting alcohol with a pivaloate group. Now—with the new leaving group in place—the key radical cyclisation sequence was re-attempted. Amazingly, when the group treated the pivalote ester with 2.5 equivalents of samarium diiodide in THF/DMPU they now obtained the desired cyclised product in 80% yield—and as an 8:1 mixture of diastereomers, in favour of the desired product.
With the key step now behind them, all that remained to complete the natural product was removal of the unwanted methyl ester, conversion of the TBS-protected secondary alcohol to the corresponding ketone, and installation of an exo-methylene group adjacent to it; however, in the presence of the molecule’s other functional groups this simple sequence of transformations proved to be far harder than expected. Heating the compound with lithium chloride in DMSO successfully effected removal of the unwanted ester via Kraptcho decarboxylation, and the TBS protecting group was removed by treatment with HCl in methanol. Reoxidation of the exposed secondary alcohol under Dess–Martin conditions then set the stage for methylenation. Unfortunately the lactam α-position proved to be more reactive than that of the ketone, making selective reaction at the desired site impossible, but the group were able to side-step this issue by protection of the lactam as the corresponding silyl ketene acetal. Methylenation next to the ketone was then accomplished by alkylation with Eschenmoser's salt, quaternisation of the resulting amine with iodomethane, and elimination under basic conditions to give the natural product.
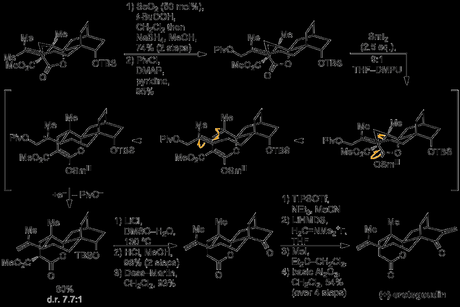
With the synthesis completed the group were able to assign the absolute stereochemistry of the natural product as the antipode of the material that they had produced, and are now working on the synthesis of other members of the family. Good job!
Miscellanea
- Interestingly, although crotonic acid was first isolated from—and named after—croton oil, it was later found that the initial structure had been misassigned and that it is in fact tiglic acid that's a major hydrolysis product of the stuff. There's actually no crotonic acid in croton oil, despite the etymological link.
- That said, for another very recent example of the use of enzymatic preparation of a chiral starting material check out this synthesis of ent-hydromorphone from T. Hud. last week!
- The award winning Merck Codexis route—detailed in this Science paper, and the accompanying In The Pipeline post—springs to mind. For now, lavishing this kind of time and money on optimising a single step to perfection is something that can really only happen in industry, but I think we’ll start to see a lot of more it as the bioengineering of enzymes becomes easier and cheaper. If you’re interested, there’s a great review on such “third wave” bioengineering in Nature, 2012, 485, 185–194.
- I have absolutely no idea why they chose to remove a t-butyl ester in this fashion; in my experience, these decarboxylate much better under mildly acidic conditions (e.g. just pour your crude reaction mixture into 2 M hydrochloric acid and stir it for half an hour at room temperature). I can see how acid might not go well with the side chain, but if that’s the case, why not just use an ethyl or methyl ester as these are removed way more easily under Krapcho-type conditions? I guess they probably have a reason, but I’m not totally sure what it could be.
- If you’re thinking that such extreme reduction could have been minimised by omitting the ethanol proton source, then you’re right in theory, but the group actually found that in reality they just got total decomposition when they tried this. This interesting observation was relegated to the paper’s SI.